Chapter 7: Design of Charging Infrastructure
Chapter Overview
In this chapter, we narrow down our focus on the infrastructure and investment needs for electric transportation. We will discuss the charging infrastructure planning, design, and operations process; specifically, where the chargers would be placed, how those are used, and who will be using these chargers. We will cover both light-duty and heavy-duty electrification needs. In addition to conductive charging, we will discuss the inductive charging process and discuss the design of wireless charging facilities.
Chapter Topics
- Charging Methods in EVs
- AC and DC Charging in EVs
- The Need for Charging Infrastructure
- Charging Station Location
- Smart Charging and ICT Aspects of Electric Charging
Learning Objectives
At the end of the chapter, the reader should be able to do the following:
- Classify the charging infrastructure based on power requirement, vehicle type compatibility, flexibility, and systems resilience.
- Locate chargers based on the ways the chargers are used and the people using the chargers.
- Estimate the equity and environmental justice implications for a given charging infrastructure scenario.
- Summarize the policy pathways to integrate charging infrastructure at the federal, state, and local levels.
- Analyze and design transportation and charging infrastructure in alignment with expanding electrification needs
Charging Technologies for EV
Fast, reliable and safe charging possibilities are required in order to help the roll-out of electric mobility. This problem can be considered as a ‘chicken and egg’ problem. Drivers will not consider the electric mobility reliable and comfortable unless there are charging facilities in predictable ranges. In contrast, the investors of the charging infrastructure would expect quick and regular income after the installation, but it will be probably a mid-term process. It should be noted that this trend can be considered similar to the relationship between the roll-out of the gasoline cars and the petrol stations almost a century ago. When the vehicles in the fleet do not have enough energy left in the battery or if the range is not sufficient to cover a journey, the vehicles are quickly charged to complete the trip. On the other hand, the vehicles can be charged at a low power when they are parked overnight. Besides this, swapping of batteries can provide a unique opportunity to get a full battery pack in minutes, similar to the short fueling times of gasoline cars.
And now, let’s dive straight into the content. You will learn about various EV battery charging methods like AC charging, DC charging, wireless inductive charging, and battery swap. The module will conclude with smart charging and Vehicle-to-Grid (V2G) and an introduction to ICT aspects of EV charging. Hope you have a successful learning experience!
Charging Methods in EVs
There are different ways to charge the EV battery. In all these methods power from the conventional AC grid is directly or indirectly converted from AC to DC to charge the EV battery. In the Introduction course, we looked at AC and DC charging of EV. Besides this, EVs can be charged by wireless inductive charging and by battery swap technology. Watch this lecture by Pavol Bauer to get an overview of the various EV charging methods!
As explained in the lecture, the three common ways to charge an EV are:
- Conductive charging – AC and DC
- Inductive charging – Static and Dynamic
- Battery swap technology
The physical location of the components for converting the power supplied by the grid to that required by vehicle battery can be categorized as onboard and offboard chargers. Onboard chargers are located within the vehicle, and the size and power rating are constrained by the available space within the vehicle. Off-board chargers are located outside the vehicle, and this setup provides more flexibility in terms of the power that can be delivered. Both classes of charging devices must contain control circuits and communicate in real-time with the vehicle battery. This is to ensure that the battery is charged in an optimum way, avoiding any damage to the battery through overcharging. AC charging uses an onboard charger while DC and battery swap use an off-board charger. In case of an inductive charger, a combination of both an onboard and off-board charger are required.
Conductive Charging
This is the most common charging method right now and it has 2 categories: AC and DC charging.
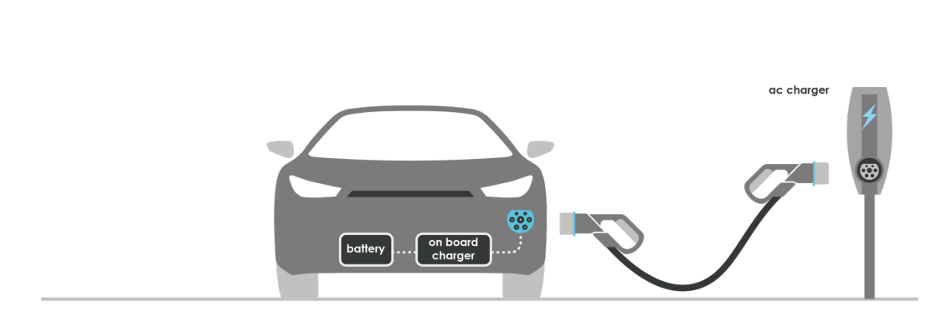
The advantages of this method are:
- The battery can be recharged anywhere using the AC grid and the onboard EV charger.
- The EV charger can easily communicate with the Battery Management System (BMS) and no additional power electronic converters are needed in the EV charger. This leads to a higher performance and lower cost.
And the disadvantages are:
- AC power has to be converted into DC power in the car, and there is a limitation of the power output for AC charging due to size and weight restrictions of the onboard charger.
- AC charging needs relatively long time due to the relatively lower charging power.
DC charging is suitable for high power EV charging, and the power output of fast charges is limited only by the ability of the batteries to accept the charging power.
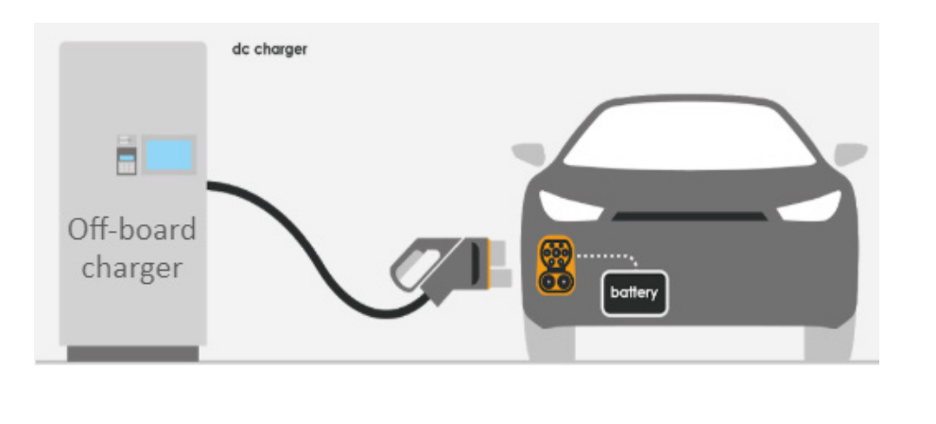
The advantages of this method are:
- It can be designed with either a high or low charging rate, and is not limited in its weight and size.
- DC charging with high power requires low charging time.
And the disadvantages are:
- Higher investment for installation of the charger when compared to AC charging.
- Adverse impact on power system: high power demand on the grid esp. at peak hours.
- Since the off-board chargers and the BMS are physically separated, reliable communication is important to ensure correct charging conditions.
Inductive Charging
The main idea behind inductive charging is the use of two electromagnetically linked coils. The primary coil is placed on the road surface, in a pad-like construction linked to the electricity network. The secondary coil is placed on the vehicle, ideally on the bottom or top of the car. The 50Hz AC power from the grid is rectified to DC and is then converted to a high-frequency AC power within the offboard charger station. Then this high-frequency power is transferred to the EV side by electromagnetic induction. The coils on the car convert this high-frequency AC power back to DC to charge the EV using the onboard charger.
The advantages of this method are:
- Convenience
- Suitable for self-driving cars
And the disadvantages are:
- High investment
- Limited space & weight of charge pads
- Misalignment tolerance between the vehicle and the charge pad
- Power losses and relatively lower efficiency than conductive charging
- Electromagnetic radiation exposure
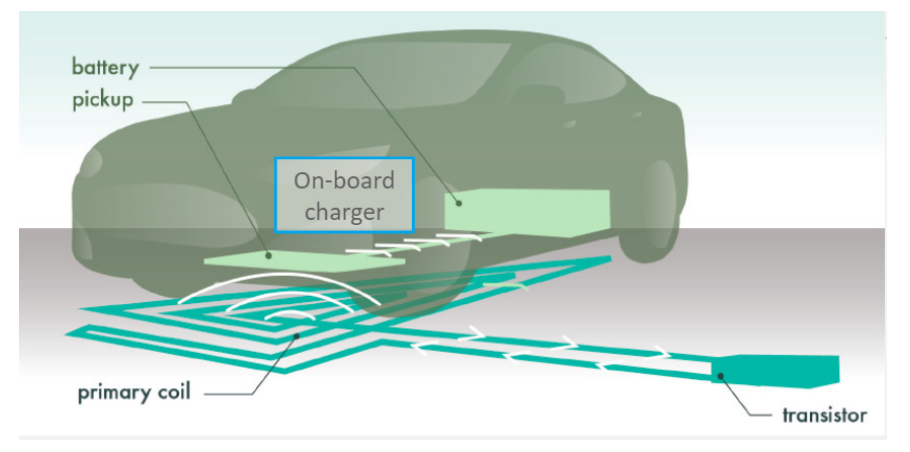
The other way to charge a car wirelessly is called dynamic charging. The coils connected to electric cables which used to provide the power are buried in the road. The coils emit an electromagnetic field that is picked up by vehicles driving over them and converted into electricity to charge the cars.
Advantages:
- Low stand-in charging time
- Low battery DoD
- Smaller battery size
So far the dynamic inductive charging is still in the experimental stage because there are many challenges to standardize it. The challenges are:
- The high cost of investment
- Foreign objects, coil structure changes and coil misalignment on the road
- Applicability of different car types and universal coil type selection
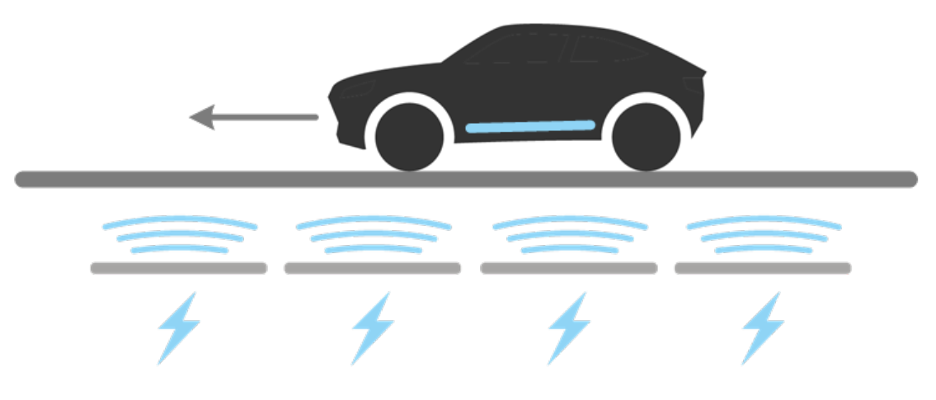
Battery Swap
The third method of EV charging is battery swap. It works on the basis of switching out the depleted battery and replacing the same with a full battery. The process involves driving into a battery switching bay and an automated process will position the vehicle, switch out the current battery and replace it with a fully charged battery. The depleted batteries are charged in the station for later deployment. The system works on the business concept that the EV user owns the vehicle and not the battery. Battery swap requires a foolproof way to estimate the batteries state of health to check for its usage pattern and to ensure that only authorized vehicles and charging stations can charge it.
The advantages of this method are:
- No range anxiety
- Quick and easy refilling like a combustion engine car tank
- Longer charging times available for the EV battery compared to fast DC Charging
The main challenges to this method are:
- The requirement of standardized battery interface across multiple car manufacturers.
- Consumer acceptance of not owning a battery and having to change the vehicle battery.
Comparison of EV Charging Methods
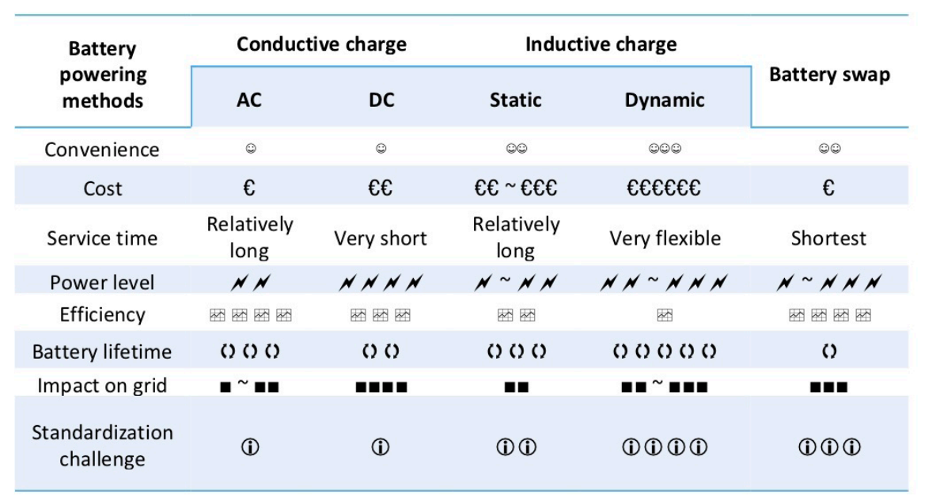
Let’s have an overall comparison of all these battery powering methods. From this table, we can see the overall comparison of all battery powering methods.
- It can be observed that the dynamic inductive charging is the most convenient charging method but also the most expensive. Even if the static inductive is cheaper compared to the dynamic one, the average cost of inductive charging is higher than any other method. Dynamic inductive charging has the most flexibility as the car can be charged at any time when on the way and do not need to stop by the service point.
- To power the battery, the battery swap method needs the shortest serving time. For all charging methods except battery swap, the serving time is highly related to the power level. In this case, the DC conductive charging method has the highest power capacity among all the methods.
- There are many factors that impact the efficiency, e.g. the number of power converters and their types, the charging power, and the charging methods. From the table, we can see that, in general, the conductive charging method has higher overall efficiency than inductive charging. It is because the power conversion process using an air gap is less efficient than direct power transfer using cables. Further, the efficiency of inductive charging reduces as a result of the misalignment between the sending and receiving charge pads.
- The battery lifetime is depending on many factors, for example, the charging power (C-rate) and the DOD. The battery lifetime in DC charging has the lowest lifetime because the charging power and hence the corresponding C-rate are the highest. Further, typically at fast charging stations, people want to charge their batteries as much as possible for long distance trips increasing the depth of discharge as well. In contrary, batteries operated with dynamic inductive charging method has the longest lifetime expectations because the batteries can be charged/discharged with small DOD.
- From the perspective of grid impact, the DC charging method has the most significant impact since it has the highest power level. Besides, the battery swap could also have a high impact on the grid if the charging powers are high as well.
- Finally, considering standardization challenge, the dynamic inductive charging and the battery swap are faced with the most difficult challenges. It is because both methods require standardization between car types, battery size, power level and even shape.
AC Charging of EVs
AC charging using the onboard charger of an EV is the most popular and simplest means of charging an EV today. Large power plants produce alternating current (AC) power which is transmitted over long distance transmission lines to our homes. Most of our homes get AC power supply from the grid and a large number of the appliances we use in our homes also run on AC power. The EV battery as we learnt earlier requires direct current (DC) power. The question that arises is how do we charge electric vehicles with AC power from the grid? Is AC charging of EVs done the same way all over the world? If not, what are the differences? And finally, the question which is most important for an EV user: how long does it take to charge your EV with AC power? Find out the answers to those questions in this lecture by Gautham Ram Chandra Mouli on AC charging of EVs.
AC charging allows EVs to be charged by using inexpensive AC charging stations which feed AC power directly from the grid to the car. The nature of AC, that is, single phase or three phase, voltage level and grid frequency may vary from country to country. AC charging uses an onboard charger to convert AC power from the conventional AC grid to direct current or DC power to charge the traction battery. Cars have a standardised vehicle inlet, and a charging cable is used for connecting the vehicle connector to the infrastructure socket of the AC charging station. The onboard charger needs to be light (typically less than 5 kg) and compact due to the limitation of allowable payload and space in the EV and the PHEV. The drawback of this charger is the limitation of the power output because of size and weight restrictions.
AC Charger Operations
When the charging station and the EV are first connected, the charge controller in the station communicates with the EV. Information regarding the connectivity, fault condition and current limits are exchanged between the charger and the EV.
- When the AC power is provided to the EV, the onboard charger has a rectifier that converts the AC power to DC power. Then, the power control unit appropriately adjusts the voltage and current of a DC/DC converter to control the charging power delivered to the battery.
- The power control unit, in turn, gets inputs from the Battery Management System or the BMS for controlling the battery charging.
- Apart from this, there is a protection circuit inside the onboard charger. The BMS triggers the protection circuits if the battery operating limits are exceeded, isolating the battery if needed.
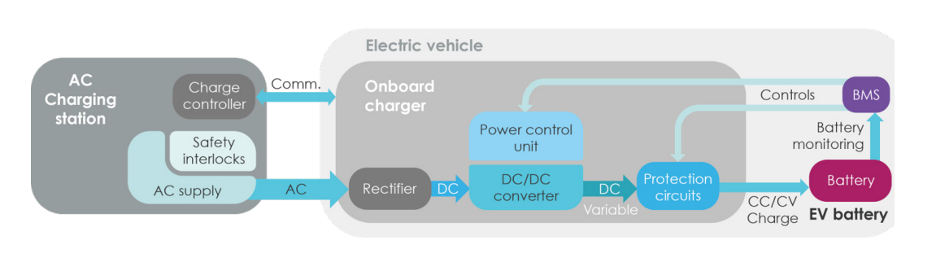
AC Charging -Types
The EV industry has not agreed on one specific AC connector, so depending on the car brand and country, the connector varies in shape, size and pin configuration. One of the main reasons is the difference in AC voltage and frequency. Generally, an AC connector has two or more larger pins to transmit power, and some smaller pins for communication. Four types of AC connectors are used worldwide, namely:
- The Type 1 connector, which is mostly used in USA & Japan.
- The Type 2 connector, which is mostly used in Europe, including those of Tesla cars.
- The Type 3 connector, used in Europe but is being increasingly phased out by Type 2 connectors.
- The proprietary connector used by Tesla for its cars in the USA.
- China has its own standard for AC charging, which is similar to Type 2 connectors.
DC charging of EVs
Range anxiety is the fear that the electric vehicle does not have enough capacity to travel anywhere that is far away. This means no EV road trips, no leisurely drives through the mountains, potentially even no cross-city travel. Yes, EV range is continuing to grow, but it is nowhere near the range of its gas-powered equivalent and the charging times are still relatively longer.
Mitigating this issue is DC fast-charging. They can charge many electric cars to 80-percent capacity in around 30 minutes, though charging rate slows down significantly after that to avoid damaging the battery pack. The tradeoff is that DC fast-charging stations are more expensive to install and operate. Large scale adoption of electric cars is possible with higher charging powers and more fast-charging stations. This allows the car makers to use smaller battery packs, thus reducing the cost of an EV’s most expensive component. Hence, could be a possible solution for the range anxiety.
We know that fast charging is quite attractive in the sense of high power charging with short charging times. But how does a DC fast charger work? What are the different DC charging systems around the world? Gautham Ram Chandra Mouli will be answering these question for you in this lecture. Find out now!
The Need for Charging Infrastructure
The usage of electric vehicles (EVs) has steadily increased over the past decade (Burnham et al., 2017) with the total number of EVs on the road in the United States (US) surpassing 1.3 million as of October 2019 (Edison Electric Institute, 2019). Despite the recent growth in the EV market, EVs still represent a small proportion of light-duty vehicles in the US and across the world, with conventional gasoline-powered vehicles (GVs) representing the largest proportion. Given EVs (i) produce zero tailpipe emissions while GVs produce a variety and high-volume of harmful local pollutant emissions and (ii) EVs can be powered by renewable energy sources with minimal greenhouse gas emissions while GVs can only utilize greenhouse gas emitting fuel, the potential social and environmental benefits of converting the light-duty vehicle fleet from predominantly GVs to predominantly EVs are significant.
One of the main factors limiting the growth of EVs is the limited public and commercial EV charging infrastructure throughout the US and the world. A specific shortcoming of existing EV charging infrastructure is the lack of ‘fast’ chargers, also known as Level 3 chargers. CHAdeMO (2020) suggests a strong positive correlation between the number of fast chargers installed in cities in Japan and the number of EVs sold in those cities, indicating an important connection between EV growth and fast-charging infrastructure. Level 3 chargers are 8 to 12 times more efficient than Level 2 ‘slow’ chargers. While Level 1 and Level 2 chargers are often used for at-home or at-destination (e.g., the workplace or shopping center) charging, Level 3 fast-charging enables en-route (or mid-trip) charging. Moreover, Level 3 charging is preferable for quick charging, long distance travel, and emergency charging, where charging time is a critical concern (Jabeen et al., 2013). Fast-charging services are also beneficial for households living in high-density residential buildings where home charging may not be available. The dearth of fast-charging facilities in the US is compounded by the shorter driving distances and longer recharging times of EVs compared to. In fact, ‘range anxiety’ from shorter driving distances between charges as well as the (in)ability to recharge batteries during travel are major obstacles for EVs in the consumer market (Egbue and Long, 2012). In 2019, the total number of EV charging stations in the US reached around 28,400 with nearly 94,000 outlets (U.S. Department of Energy, 2020). The state of California, which is the largest EV market in the US, has more than 6900 stations and more than 29,500 outlets (U.S. Department of Energy, 2020). However, only 14.6% of all charging stations in the US provide Level 3 fast-charging service and the current number of Level 3 fast-charging stations cannot fulfill the demand for fast charging throughout most of the country (U. S. Department of Energy, 2020).
Potential problems associated with this supply–demand imbalance include long waiting times at fastcharging stations and/or long detour distances to find an EV fast-charging station. Clearly, there is a significant need to invest in and expand EV fast-charging infrastructure capacity in order to support the growth of the EV market. This need is well known by both governmental agencies looking to support EVs and car manufacturers who are selling EVs. In addition to strategic planning, there is also a need to manage the EV fast-charging capacity efficiently at individual stations and across a region of fast-charging stations. Given the expected rapid growth rate of EVs over the next decade or two, there are likely to be imbalances between the supply of fast-chargers and the demand for fast charging, even as public sector and private companies work to expand fast-charging capacity. Managing supply and demand at the individual station level and across a regional system of fastcharging stations is important for minimizing user wait times at fast-charging stations and utilizing existing capacity effectively at fast-charging stations. Moreover, when demand outpaces supply in an area, demand-responsive pricing-based management strategies can generate revenue to support the funding and financing of additional fast-charging capacity to balance supply with demand in the long run.
Electric vehicles vs. Gasoline vehicles
GVs dominate the personal vehicle market and are a relevant reference point to compare with the service quality offered by EVs. There are several key differences between EVs and GVs in terms of recharging and refueling that have implications for the planning and management of EV fast-charging infrastructure. First, in one important way, recharging an EV is more flexible than refueling a GV. Unlike GVs that are almost exclusively refueled at dedicated gasoline refueling stations, an EV can be recharged in at least three different types of places—at a dedicated EV recharging station, in a general parking lot with installed chargers (e.g., at workplaces, shopping centers, etc.), or at the EV owner’s house. Home-installed chargers are typically Level 1 or Level 2 chargers while Level 3 chargers are only available at dedicated charging stations because of power supply and safety concerns. Home- and workplace-installed ‘slow’ chargers are mainly used for destination charging, while public fast-chargers aim to facilitate en-route charging, particularly for long-distance trips. Second, the average range of an EV when fully charged is typically shorter than that of a similar GV. Although the average travel range of EVs has steadily increased in recent years, range-anxiety is still a concern for current and potential EV owners. The average range for an EV sedan is around 200 miles, which is only one-half to two-thirds the range of a typical GV sedan. This range issue has implications in terms of the frequency with which EV owners need to recharge and also the spatial distribution of EV fast-charging stations needed to support (long-distance) EV travel. Properly sizing and siting fast-charging stations is mainly a planning level challenge; however, these stations also need to be managed efficiently, particularly when demand outpaces supply (in certain areas, during certain times of the day). Third, and most importantly from a real-time management perspective, the recharging time of an EV is considerably longer than the refueling time of a GV. Even with a 50 kW Level 3 fast charger, to recharge an EV from empty to 80% capacity takes thirty minutes to an hour. Hence, if an EV does require the use of an EV fast-charging facility, the vehicle may occupy a charger at the station for a significantly longer period of time than a GV does at a gasoline station. Moreover, if an EV user goes to a recharging station with a queue, he/she may have to wait an extended period of time to even begin recharging. When a limited number of EV fast-charging stations are constructed along a high-volume corridor, a spike in local demand may create queues at one or more EV recharging stations, thereby inconveniencing EV users who need to recharge but do not have many alternative fast-charging stations nearby.
EV charging technology
The types of chargers usually found in public EV charging stations in North America are the J1772, the CCS (Combined Charging System), the CHAdeMO (abbreviation of “Charge de Move”), and the Tesla Supercharger. The first one is a Level 2 charger, while the other three include Level 3 Direct Current Fast Charging (DCFC) chargers (they are also capable of lower level charging). Table 1 summarizes EV charger types from Level 1 to Level 4. As mentioned previously, Level 3 chargers are 8 to 12 times more efficient than Level 2 chargers. Level 4 charging—also called Extreme Fast Charging (XFC)—is not currently available. Level 4 chargers could further improve charging efficiency and narrow the current refueling/recharging time difference between EVs and GVs.
Table 7.2 shows that compared with Level 2 chargers, Level 3 and 4 chargers have considerably more output power and therefore require higher voltage. The large loads from fast-charging stations will ultimately become a burden for electric grids and may cause system failures in the worst-case scenario. Studies have been done on both supply and demand sides of EV charging to avoid the situation. On the supply side, researchers propose methods of upgrading infrastructure and integrating fast-charging stations into the grid (Aggeler et al., 2010; Dharmakeerthi, Mithulananthan, & Saha, 2012; Guo, Deride, & Fan, 2016; Burnham et al., 2017; Chen et al., 2017; Iyer et al., 2018). On the demand side, researchers use multiple approaches to model demand and propose control schemes (e.g. pricing) to balance the spatial and temporal demand for charging (Cao et al., 2012; Chen & Frank, 2001; Flath et al., 2014).
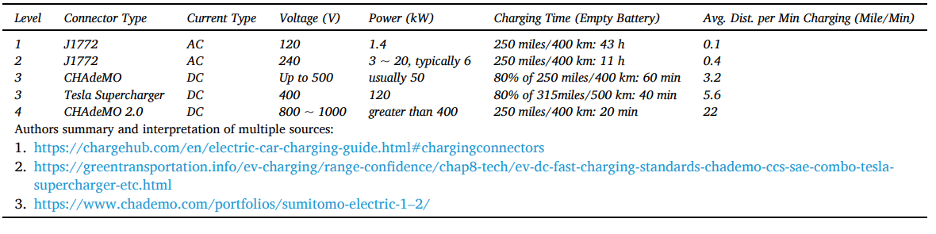
Charging Station Location
EV charging demand and station choice behavior
Understanding and effectively capturing spatial–temporal demand patterns and EV user behavior is a crucial component for developing models to support the real-time management of a system of EV fast-charging stations. The relevant behavioral attributes in much of the EV station choice literature include, charging cost (Jabeen et al., 2013; Wen, MacKenzie and Keith, 2016; Daina, Sivakumar and Polak, 2017; Ge, MacKenzie and Keith, 2018), distance or detour distance to charging stations (Daina, Polak and Sivakumar, 2015; Sun, Yamamoto and Morikawa, 2016; Yang et al., 2016), as well as EV state of charge (SOC) and/or charging time (Sun, Yamamoto and Morikawa, 2015; Yang et al., 2016; Xu et al., 2017; Pan, Yao and MacKenzie, 2019). The model in the current study considers the following factors: charging price at each EV fast-charging station, detour distance for an EV driver to visit each station, and expected wait time at each station.
The behavioral modeling approaches in the literature include the multinomial logit (MNL) (Jabeen et al., 2013; Daina et al., 2017), the mixed logit (Sun, Yamamoto and Morikawa, 2015, 2016; Xu et al., 2017) and the nested logit (Yang et al., 2016), which are all discrete choice modeling approaches. Other modeling approaches for charging behavior include those by Kang & Recker (2009) who use an activity-based model and by Hu, Dong, & Lin (2019) who model choices based on cumulative prospect theory. In Jabeen et al. (2013), the charging choice is whether to charge at home, at work, or at public stations. The three alternatives are independent, as such the study employs the MNL model. The mixed logit model is appropriate when there is unobserved heterogeneity among users, differences in tastes, and/or when using panel data (Sun, Yamamoto and Morikawa, 2015). In Yang et al. (2016), the choice of charging is integrated with routing and the authors employ a nested logit model for route and charging choices. See Train (2009) for an overview of discrete choice models and their applications.
Management and pricing of EV Fast-charging stations
This section reviews literature associated with managing EV charging stations via using different pricing strategies. One important application of pricing schemes is to manage and balance EV charging demand. These management schemes include Price Based (PB) and Demand Response (DR) Programs in electricity markets (Albadi and El-Saadany, 2008).
PB management programs are usually based on Time-of-Use (TOU) pricing or real-time pricing (RTP). TOU pricing involves charging users separate prices for peak and off-peak hours in order to shift loads from peak to non-peak periods. TOU prices are usually provided to users in advance; therefore, they cannot be used to respond to within-period demand stochasticity. TOU related studies include Cao et al., (2012) and Bayram et al. (2015).
RTP involves dynamic price changes based on current demand levels. Chen & Frank (2001) study general service price adjustments based on the state of queues. Their study assumes that firms are capable of changing prices and that customers adjust behaviors based on queue states. Their results indicate that firms can increase surpluses while social welfare increases, under homogeneous customer behavior. Chen et al. (2017a) design an RTP mechanism based on an automatic DR strategy for Photovoltaic-assisted charging stations. Luo, Huang and Gupta (2018) consider a case for stochastic dynamic pricing where renewable resources and energy storage are integrated to charging suppliers.
The management approach in this paper involves RTP under which fast-charging station prices are dynamically adjusted based on current demand levels, i.e. DDRPA schemes. It extends the setting in Chen & Frank (2001) to a larger area with multiple EV fastcharging stations managed by the same entity. This paper describes schemes that enable heterogeneous pricing across stations based on the current state of each individual station, which is unlike the spatial or area pricing schemes used in earlier literature.
It is worth noting that several studies in the literature model the dynamic interplay between the electric grid power supply and EV charging station energy acquisition for management purposes (Dharmakeerthi, Mithulananthan and Saha, 2012, 2014; Sbordone et al., 2015; Khan, Ahmad and Alam, 2019). Future research may combine the user-and-EV station dynamic models and management strategies in the current paper, with the EV station-and-power grid dynamic models and management strategies in the literature.
Smart Charging
The unique aspect of EV charging is that the charging process can be controlled in time. For example, if an EV that needs 20kWh is connected for 8h to a 10kW charger, then upto 80 kWh can be delivered. But since only 20 kWh is needed, the charging can be done either at a lower power, moved in time to occur for any 2 hours within the 8 hours or done in parts. This flexibility in EV charging can be used for various applications such as increase the use of renewables or lower the charging costs. In the future, electric vehicles can even operate as a backup for the grid on a relatively large scale. In case of a short duration failure, EVs can be connected to the grid, to our homes or to loads and can be controlled to provide emergency power via Vehicle to Grid (V2G) charging. Pavol Bauer will teach you this concept in his lecture below.
ICT Aspects of Electric Charging
Charging electric cars is part of an evolving ecosystem with many different components and stakeholders that must be able to exchange information automatically. For example, charging systems today require communication between the EV user, his/her charging card, payment portal, charge point operator to do a simple AC charging of an EV in the public terrain. With the advent of smart charging, this ecosystem is expanded even further to include players like the distribution system operator, the transmission system operator and the balance responsible party. In this video, Auke Hoekstra will explain how you can use a range of ICT protocols to do just that.
Case study: ABB EV charging infrastructure
Now that we have learnt about all the EV charging concepts, let us now look at how it is done in the real world. For this, we will have a case study with ABB EV Charging Infrastructure (http://new.abb.com/ev-charging) and meet with Crijn Bouwman, Vice President of Product Management.
ABB specializes in DC fast chargers ranging from 50kW to 150kW (Chademo, Combo, GB/T) with modular chargers going upto 400kW. They also offer AC charging solutions for electric cars and overnight and opportunity chargers for busses and trucks.
Key Takeaways
- There are various charging methods for EVs. The three common ways are conductive charging, inductive charging and battery swap.
- Conductive charging is the most common charging method right now and it has two categories: AC and DC charging. The second method is inductive charging and consists of two types: static and dynamic charging.
- There are several benefits of smart charging and it has huge potential: EV charging can be controlled based on renewable generation, energy prices, grid loading and how the EV can be used as a storage and as an emergency power supply.
- Climate change in turn affect how a transportation system is planned, designed, and operated in the future.
Self-Test
Glossary: Key Terms
CCS (Combined Charging System): The Combined Charging System (CCS) is a standard for charging electric vehicles. It can use Combo 1 (CCS1) or Combo 2 (CCS2) connectors to provide power at up to 350 kilowatts (kW) (max 500 amps).
Direct Current Fast Charging (DCFC): DC fast chargers convert AC power to DC within the charging station and deliver DC power directly to the battery.
Extreme Fast Charging (XFC): XFC is defined as the ability to replace at least 80% of the vehicle battery capacity in 15 min or less, or to replenish range at a rate of ∼20 miles per minute of charge.
Range Anxiety: Range anxiety, a term commonly associated with electric vehicles (EVs), refers to the apprehension and worry experienced by EV owners about the adequacy of their vehicle’s battery charge to complete a journey or the availability of charging stations along the way.
Time-of-Use (TOU): Time-Of-Use (TOU) pricing is a variable rate structure that charges for energy depending on the time of day and the season the energy is used. With TOU rates, your bill will be determined by both when you use electricity and how much you use.
Vehicle-to-Grid (V2G): V2G, technology is smart charging tech that allows car batteries to give back to the power grid.
Media Attributions
Figures
- Figure 7.1: Conductive Charging- AC by Electric Cars: Technology Electric Cars: Technology is licensed under CC BY-NC-SA 4.0
- Figure 7.2: Conductive Charging- DC by Electric Cars: Technology is licensed under CC BY-NC-SA 4.0
- Figure 7.3: Inductive Charging- Static by Electric Cars: Technology is licensed under CC BY-NC-SA 4.0
- Figure 7.4: Inductive Charging- Dynamic by Electric Cars: Technology is licensed under CC BY-NC-SA 4.0
- Figure 7.5: AC Charging Operations by Electric Cars: Technology is licensed under CC BY-NC-SA 4.0
Tables
- Table 7.1 is licensed under CC BY-NC-SA 4.0
- Table 7.2: Summary of Common Level 1 to Level 4 Charging Facilities in North America is licensed under CC BY 4.0
Videos
- Video 1: Introduction to Charging Methods of EVs is available for free via http://www.online-learning.tudelft.nl ©️ TU Delft, released under a CC BY NC SA license: https://creativecommons.org/licences/…
- Video 2: AC Charging of EVs is available for free via http://www.online-learning.tudelft.nl ©️ TU Delft, released under a CC BY NC SA license: https://creativecommons.org/licences/…
- Video 3: DC Charging of EVs is available for free via http://www.online-learning.tudelft.nl ©️ TU Delft, released under a CC BY NC SA license: https://creativecommons.org/licences/…
- Video 4: Smart Charging and V2G is available for free via http://www.online-learning.tudelft.nl ©️ TU Delft, released under a CC BY NC SA license: https://creativecommons.org/licences/…
- Video 5: Charging of Electric Vehicles ICT Aspects of Electric Charging is available for free via http://www.online-learning.tudelft.nl ©️ TU Delft, released under a CC BY NC SA license: https://creativecommons.org/licences/…
- Video 6: Case Study ABB is
References
- Bending the Curve: Climate Change Solutions by Ramanathan, V., Aines, R., Auffhammer, M., Barth, M., Cole, J., Forman, F., et al. is licensed by the following creative commons license: CC BY-NC-SA 4.0
Attributions
- Bending the Curve: Climate Change Solutions by Ramanathan, V., Aines, R., Auffhammer, M., Barth, M., Cole, J., Forman, F., et al. is licensed under CC BY-NC-SA 4.0
- Dynamic modeling and real-time management of a system of EV fast-charging stations by Yang, D., Sarma, N., Hyland, M., & Jayakrishnan, R is licensed under CC BY 4.0 Deed
- Electric Cars: Technology by TU Delft OpenCourseWare is licensed under a Creative Commons Attribution-NonCommercial-ShareAlike 4.0 International License.
V2G, technology is smart charging tech that allows car batteries to give back to the power grid
Range anxiety, a term commonly associated with electric vehicles (EVs), refers to the apprehension and worry experienced by EV owners about the adequacy of their vehicle's battery charge to complete a journey or the availability of charging stations along the way.
The Combined Charging System (CCS) is a standard for charging electric vehicles. It can use Combo 1 (CCS1) or Combo 2 (CCS2) connectors to provide power at up to 350 kilowatts (kW) (max 500 amps).
DC fast chargers convert AC power to DC within the charging station and deliver DC power directly to the battery.
XFC is defined as the ability to replace at least 80% of the vehicle battery capacity in 15 min or less, or to replenish range at a rate of ∼20 miles per minute of charge.
Time-Of-Use (TOU) pricing is a variable rate structure that charges for energy depending on the time of day and the season the energy is used. With TOU rates, your bill will be determined by both when you use electricity and how much you use.