5 Transportation and Mobility Modes
CHAPTER OVERVIEW
This chapter introduces transportation modes by defining and categorizing them based on whether they primarily serve passengers or freight and exploring the concepts of multimodal and intermodal approaches to transportation. It includes a section on modal share, an important transportation planning statistic revealing a city or region’s predominant and minor forms of mobility. Factors influencing travel behavior and mode choice are examined to shed light on the reasons behind the relatively low usage of public transportation and active transportation (such as walking and cycling) in the United States. The chapter also discusses and illustrates passenger and freight intermodal transportation systems before briefly touching upon emerging smart mobility trends within the context of intermodal transportation.
CHAPTER TOPICS
- Defining Transportation Modes
- Multimodal and Intermodal Systems
- Modal Share in the U.S.
- Factors Affecting Mode Choice
- Transit Modes
- Intermodal Transportation
- Conclusion
- Quiz
- Glossary
- Acronyms
LEARNING OBJECTIVES
Learning Objectives
-
Identify the main modes of passenger and freight transportation in the US.
-
Differentiate, providing examples of multimodal vs. intermodal approaches to transportation.
-
Define modal split and identify at least three urban areas with relatively large shares of transit ridership providing reasons for this deviation from the national modal share figures.
-
Identify and explain the factors transportation research has shown to be associated with travel behavior and mode choice in the US.
-
Discuss the role of public-private partnerships in smart intermodal solutions identifying the modes being combined in some American cities along with their transit service context.
INTRODUCTION TO TRANSPORTATION MODES
Transportation mode refers to the method of mobility and encompasses various means of transporting people or goods. Examples of transportation modes include road transportation using vehicles like cars, trucks, motorcycles, and bicycles, air transportation via airplanes and drones, railway transportation involving passenger and cargo trains, and water transportation utilizing ferries and ships. Additionally, there are other energy transport modes, such as pipelines, cable transport, and space transport. Each mode depends on infrastructure, vehicles, transport operators, and operations. Therefore, different modes address different mobility issues. A crucial aspect of transportation planning and programming involves establishing and maintaining an efficient transportation infrastructure that supports diverse modes (“Mode of Transport,” 2022).
Understanding travel modes is vital in transportation planning for several reasons. It helps in comprehending travel behavior, anticipating future transportation requirements, identifying opportunities for active transportation such as walking, cycling, and transit, and promoting them to alleviate congestion and air pollution. Moreover, transportation modes have direct implications for health and safety, impacting community lifestyles, social equity, and economic development. Therefore, studying transportation modes is integral to transportation planning and policy, with implications for climate change mitigation, public health, safety, equity, and urban economic development.
Demand for mobility modes is influenced by various factors, including the built environment, specific features of each mode, socioeconomic factors of users, and attitudes toward mobility options. These factors play a critical role in determining the adoption, acceptance, and utilization of different transportation modes. Usability, infrastructural requirements, and environmental impacts characterize different transportation technologies. Hence, other mobility solutions can be evaluated based on various criteria (Brunner et al., 2018). For instance, walking is an active mobility mode; it must be designated and devoted to paths to be effective. Therefore, walkability can be evaluated by the presence of good-quality walking paths.
While this chapter primarily focuses on surface transportation modes related to passenger travel, it briefly touches upon freight mobility by air, rail, and water under the topic of intermodal transportation. A more detailed exploration of freight modes falls beyond the scope of this chapter. Interested readers seeking further information are encouraged to refer to alternative publications such as “Transportation as a Derived Demand: The Geography of Transport Systems” by J. P. Rodrigue (2020), published by Routledge.
Defining Transportation Mode
A transportation mode refers to the specific method used for traveling or transporting people and goods, each relying on distinct infrastructure, vehicles, operators, and operations. Except for walking, modes rely on specific mobility technologies and fuel for movement on land, air, or water. These modes vary in usability, infrastructural requirements, operator licensing, and environmental impacts, influencing the institutional, environmental, and operational deployment conditions. For example, cycling requires designated paths for safety and effectiveness, while car driving necessitates road infrastructure, operator licensing, fueling stations, traffic controls, emission controls, and policing for efficient circulation (Brunner, et al., 2018). Each transportation mode involves a complex combination of technology, infrastructure, energy, and regulations, often referred to as a “transportation regime” in policy circles and sociotechnical-systems research. Research on transportation regimes entails technology, infrastructure, regulation, user behaviors, cultural significance and market dynamics, all of which interact to maintain stability within a specific sociotechnical system. For instance, in the land passenger transportation system, the prevalence of the car mode represents a dominant regime due to the wide range of factors that reinforce it. These factors include powerful players such as the oil and automobile industries, government investments in road and highway infrastructures, as well as cultural and personal values associated with time, individual freedom of movement, and social status of car ownership (Hirshhorn et al., 2019, Hoffman et al, 2017, Sovacool and Axsen, 2018, Urry 2004). This dominance of the car has made other modes like public transit secondary transportation regimes in North America, while European countries have actively sought to challenge this dominance (Hirshhorn et al., 2019, Hoffman et al, 2017, Geels, 2018). Although this chapter primarily focuses on transportation modes’ definition, types, the concept of modal split, planning approaches, and factors affecting modal choice, it is important to recognize the sociotechnical perspective, which serves as a valuable backdrop and reminder that mobility modes must be understood within a broader context, or regime. This perspective becomes increasingly pertinent in the ongoing transition towards “smart” and other emerging mobility solutions.
Origin of the Concept
While transportation modes have existed since ancient times, a modern understanding of the concept emerged in the late 19th and early 20th centuries, when early transportation engineers recognized four primary modes: railroads, water carriers, pipelines, and horses (Walton, 2014). However, by 1929, the Regional Plan Association (RPA) of New York and its Environs acknowledged the challenges posed by vehicular traffic, with over a million motor vehicles (including automobiles, trucks, buses, and taxicabs) in circulation at a rate of 7.6 vehicles per inhabitant (RPA, 1929). The plan’s “Ways of Communication,” which addressed both pedestrian and vehicular traffic issues, proposed improvements to the railroad system (subway, trunkline transit, and suburban rapid transit), waterway system, regional highway system, and air transportation system.
In traditional transportation terminology, the five modes (motor vehicles, railroads, airlines, water carriers, and pipelines) mainly pertain to freight transportation. However, contemporary transportation planning also encompasses passenger modes (like cars, motorcycles, ground and water transit) and active transportation modes (such as cycling and walking). Some authors even suggest considering the Internet as an additional mode (Walton 2014).
MULTIMODAL AND INTERMODAL SYSTEMS
The following are two fundamental ways of looking at and discussing transportation modes with respect to their coordination and level of integration.
- Multimodal: Depending on the system, we have the option of selecting from a variety of modes for a trip. A multimodal trip can consist of any combination of modes such as, walking, cycling, riding public transit, and driving a personal motor vehicle (PMV). Example: A trip that involves traveling by train to a central station, transferring to a bus to reach a local destination, and then walking to the final destination. In a multimodal system, each mode operates independently, there is no coordination among the modes and passengers or cargo transfer between modes at specific points on their own.
- Intermodal: Intermodal systems involve the integration of multiple modes of transportation. In a sense, all intermodal systems are multimodal but include service integration by a public or private transportation agency and the existence of intermodal facilities for the transfer from one mode to the other. Example: a bus service providing bike racks, and a timetable app makes it possible for passengers to connect between the two modes. The passenger boards the bus at the bust stop, places the bicycle on the bus rack, alights and bikes to the final destination. Intermodal facilities can be for passengers or freight. For example, a bus terminal at an airport serves to transfer passengers at least between three modes, walking, bus, and air. Transfers between trucks, trains, and ships are referred to as intermodal in the freight industry and rely on containers for the intermodal transfers of goods. Figure 5.1 shows a detailed comparison of different shipping modes along with their pros and cons.
When deciding among transportation modes, shown in Figure 5.2, various factors come into play. These factors include the presence of infrastructure (e.g., sidewalks, paths, roads, rails), intermodal facilities (bus stops, terminals, ports, ferry stations, and airports), the built environment and land use patterns, urban design, and socio-economic and cultural behaviors. The degree to which these factors influence modal decisions also varies depending on the type and purpose of travel. For example, socio-economic factors may significantly affect mode choice for work-related commutes, whereas for leisure or non-work trips such as dining out or shopping, the availability of infrastructure like parking or bike paths or the urban design of the built environment could be more critical.


MODAL SHARE IN THE U.S.
Modal share, or modal split, refers to the percentage of travelers or trips utilizing a specific transportation mode in an area, city, region, or country. A significant portion of trips and travel miles occur within urban areas. According to the 2017 National Household Travel Survey (NHTS), the average length of urban trips in the US has increased over time, from around 8.89 miles in 1969 to over 10.5 miles in 2017 (Federal Highway Administration, 2017). However, these statistics primarily focus on vehicle trips. For alternative modes like walking and cycling, known as active transportation, trip distances are generally much shorter, ranging from 0.5 to 2 miles. Despite this, walking and cycling account for approximately 10% and 1% of all trips, respectively. Surprisingly, even for trips under 2 miles, which make up 40% of all trips, private cars remain the dominant mode of travel. This can be attributed to car-oriented urban patterns prevalent in the US, which have made active modes less appealing and perceived as unsafe for various travel purposes.
Similar to the impact on other transportation modes, public transit is influenced by factors such as car-oriented development patterns, which affect both the supply and demand of transit services. However, additional factors affecting public transit include the quality and availability of services, fare rates, and reliability (Erhardt et al., 2022). External factors like socio-economic and demographic trends, such as income levels, gas prices, and the COVID-19 pandemic, also play a significant role in transit ridership. For instance, the COVID-19 pandemic led to a drastic decline in transit ridership, with passenger trips dropping from 9.0 billion in 2019 to 4.5 billion in 2020, representing a 45 percent reduction. Although transit ridership is gradually increasing, it has not yet returned to pre-pandemic levels (USDOT 2023, p.1-2).
According to the Bureau of Transportation Statistics, the most common modes of travel for commuting include driving alone, carpooling, working from home, public transport, taxi, motorcycle, walking, and cycling. In 2021, the modal share for commuting trips for each of these modes was as follows: 67.82% for driving alone, 7.79% for carpooling, 17.86% for working from home, 2.46% for public transport, 1.47% for taxi, 2.2% for motorcycle, and 0.4% for walking and cycling, respectively. Table 5.1 displays the mode share in 2022 for the U.S. largest urban areas (population > 1 million). The low share of bicycle trips reflects the limited popularity of cycling as a mode of commuting in US cities. Figure 5.3 illustrates the modal split for urban trips from 2010 to 2021, showing a notable increase in teleworking during the years 2020 and 2021 due to the COVID-19 pandemic. Table 5.1 shows that in 2022 the share of working at home remained significant (> 20%) for many large cities. In the following sections, we will explore the reasons behind the lower shares of active transportation and public transit compared to driving private vehicles. Automobility has remained the predominant mode of travel in the US since the 1960s. Every year the United States. Department of Transportation. Bureau of Transportation Statistics releases a Transportation Statistics Annual Report (TSAR) which describes the transportation system of the United States in terms of the system’s performance, its contributions to the economy, and its effects on the environment (USDOT, 2023).

2022 Urban Area | Commute (minutes) | Drove alone (%) | Carpooled (%) | Public transit (%) | Bicycled (%) | Walked (%) | Worked at home (%) | Other (%) |
---|---|---|---|---|---|---|---|---|
Austin. TX | 24.7 | 59 | 7 | 1 | 0 | 2 | 30 | 1 |
Baltimore, MD | 28.1 | 67 | 7 | 4 | 0 | 2 | 18 | 2 |
Boston, MA-NH | 30.1 | 56 | 6 | 9 | 1 | 5 | 21 | 2 |
Dallas-Ft Worth-Arlington, TX | 26.7 | 69 | 9 | 1 | 0 | 1 | 18 | 1 |
Chicago, IL-IN | 30.4 | 61 | 8 | 8 | 1 | 3 | 18 | 2 |
Denver-Aurora, CO | 26.3 | 63 | 7 | 2 | 1 | 2 | 23 | 1 |
Houston, TX | 29.7 | 71 | 11 | 2 | 0 | 1 | 13 | 3 |
Las Vegas-Henderson-Paradise, NV | 24.6 | 70 | 11 | 2 | 0 | 1 | 13 | 3 |
Los Angeles-Long Beach-Anaheim, CA | 29.1 | 65 | 10 | 3 | 1 | 2 | 17 | 2 |
Miami-Fort Lauderdale, FL | 29.5 | 69 | 9 | 2 | 1 | 2 | 15 | 2 |
NY-Jersey City-Newark, NY-NJ | 35.9 | 44 | 6 | 25 | 1 | 6 | 16 | 2 |
Philadelphia, PA-NJ-DE-MD | 28.5 | 63 | 7 | 6 | 1 | 3 | 19 | 2 |
Phoenix-Mesa-Scottsdale. AZ | 25 | 63 | 10 | 1 | 1 | 1 | 22 | 2 |
Portland, OR-WA | 23.9 | 59 | 7 | 3 | 1 | 3 | 24 | 1 |
San Antonio, TX | 26 | 68 | 12 | 2 | 0 | 2 | 15 | 2 |
San Diego, CA | 25.2 | 66 | 8 | 2 | 1 | 3 | 19 | 1 |
San Francisco-Oakland, CA | 29.2 | 47 | 8 | 9 | 2 | 4 | 28 | 2 |
Seattle-Tacoma, WA | 27.6 | 56 | 8 | 5 | 1 | 3 | 26 | 1 |
Washington-Arlington, DC-VA-MD | 31.1 | 53 | 8 | 7 | 1 | 3 | 27 | 2 |
Note: From “Census Reporter, Urban Areas Profiles (ACS 2022 data),” by U. S. Census Bureau (https://censusreporter.org/search/). Public Domain. |
FACTORS AFFECTING MODE CHOICE
As the distance to a destination increases, its value or utility diminishes. Conversely, shorter distances make travel more likely, resulting in a decrease in the rate of distance decay. Distance decay refers to the spatial barriers that hinder reaching a destination from the trip’s origin and can be quantified in terms of time, cost, or trip length. The nature of distance decay varies depending on factors such as traffic congestion and road quality. The relationship between travel costs and distance decay can take different forms, including inverse distance relationships or other non-linear functions (Cheng & Bertolini, 2013). Additionally, the impact of distance is perceived differently based on income levels and trip purposes, leading to variations in the distance decay function depending on household socio-economic status and chosen mode of travel (Geurs & van Eck, 2003).
For example, data from the Metropolitan Washington Council of Governments’ 2007/08 regional traffic survey, which is part of the National Household Travel Survey, shows that short walks are common among travelers. In terms of bicycle trips, approximately 90% of them cover distances of 8 kilometers or less. Research indicates that bicyclists tend to travel longer distances compared to pedestrians and are willing to devote more time to their journeys (Kuzmyak et al., 2014).
On the other hand, factors such as automobile ownership, long commuting distances, and workforce participation are positively associated with driving, while retirement or unemployment and low income tend to be negatively associated with personal vehicle use as a commuting mode choice. Aside from trip purpose and distance, the factors influencing mode choice can be categorized into two groups: households’ endogenous factors, which include individual characteristics like household income, race, gender, education, perceptions, and attitudes, and exogenous factors, which encompass contextual and environmental elements such as the built environment, traffic conditions, weather, transportation policies, pandemics, and economic shocks.
Numerous studies in travel research have attempted to identify the determinants of mode choice, utilizing various methodologies. Traditional approaches involve stated preference (SP) and revealed preference (RP) surveys to gather travelers’ and trip information, relying on economic utility theory to predict mode choice. In contrast, newer methods leverage smart cards, mobile phones, Wi-Fi, and GPS big data collection sources, employing machine learning models to construct traveler profiles and predict their mode choice and trip characteristics (Lai et al., 2022, Lv et al., 2014).
Active Modes: Walking and Cycling
Several considerations can significantly influence the decision to engage in active travel like walking and bicycling because these modes are relatively more context-sensitive than motorized ones.
Numerous factors, including transportation mode, destination selection, and route preference, have been found to affect walking and bicycle behavior. The factors that determine the choice of active transportation modes have been examined across many disciplines spanning from the policy context of a region to built-environment conditions, social and socio-demographic factors, individual preferences, and trip-related attributes. While all these factors play a role in shaping the decision to engage in active transportation, from an urban transportation planning standpoint, the most important factors for sustaining and enhancing active transportation are:
- Built environment (land use, type of mobility network, density, and connectivity)
- Natural environment (topography, climate/weather)
- Socio-economic and demographic factors, attitudes, and perceptions (Kuzmyak et al., 2014).
As we’ll explore in the upcoming sections, each of these broad categories can have varying effects on the choice between walking and cycling as modes of travel. For example, urban areas with higher densities and walkable neighborhoods often make walking and cycling more feasible compared to low-density suburban developments, which tend to offer higher-income households more mobility options and make driving more convenient and desirable. However, with the aim of achieving equity and sustainability in transportation, urban planners strive to promote alternative modes such as public transit, ridesharing, and active transportation. This is to create an inclusive transportation system where everyone, regardless of income, race, physical abilities, or residential location, has the opportunity to travel.
Built Environment and D’s Research
Numerous studies have investigated the built environment’s influence on travel behavior. One well-known concept introduced by Cervero & Kockelman (1997) is the “three Ds,” which refers to density, diversity, and design. Later, two more “Ds” were added: destination accessibility and distance to transit. Demand management strategies such as parking supply or congestion pricing were also added as a 6th “D” and more recently, a 7th “D” for demographics was incorporated as a control variable in travel behavior studies also known as D’s research (Ewing & Cervero, 2001, 2010). The main five Ds are:
- Density refers to the measurement of a variable of interest per unit area, whether it’s population, employment, housing, office space, open space, or other land uses.
- Diversity represents the level of heterogeneity (entropy) for a variable of interest and can measure the mix of land uses, employment, housing, and nearby destinations within an area.
- Design pertains to block sizes and the layout and connectivity of streets. This includes factors such as the number of intersections within a neighborhood, the presence of sidewalks and tree canopies, street network patterns (e.g., grid or cul-de-sac), and whether streets are designed as complete streets.
- Destination accessibility is a key factor that measures how easily destinations can be reached from a specific location. Hansen’s equation is often used to calculate accessibility, considering factors like distance, time, or cost of travel between origin and destination as the resistance (impedance) factor challenging ease of access.
- Distance to transit evaluates the convenience of making transit trips based on the shape of the built environment. It can be measured in Euclidean or network-based distances, considering the proximity to rail stations or bus stops, or determined by the density of transit stations or routes (Ewing & Cervero, 2010)
However, by 2018, some dissenting researchers criticized “Ds” research, arguing that the focus on “design” factors was catchy but misleading. They questioned the use of density as a predictor without a clear theory or explanation of how density influences travel behavior (Handy, 2018). Additionally, they highlighted the problem of treating the various “Ds” as independent factors when they are highly interrelated, leading to estimation errors in predicting travel behavior. As Handy (2018) suggests, in the excerpt of Box 5.1, focusing on accessibility rather than the “Ds” may provide a more sensible approach for researchers, practitioners, and the public alike.
Box 5.1 Critique of the D’s
Enough with the “D’s” Already — Let’s Get Back to “A”
by Susan Handy, Transfers Magazine, Spring 2018
When Robert Cervero and Kara Kockelman published their highly-cited article “Travel demand and the 3Ds: Density, diversity, and design” in 1997, they seemingly changed the transportation planning discourse forever. The idea of characterizing the built environment using three measures that happen to start with the letter D was catchy and catch on it did.
By 2010, the 3Ds had grown to seven with the addition of “destination accessibility” (what I would call regional accessibility), “distance to transit,” “demand management,” and “demographics” (a category of control variables, not a characteristic of the built environment).
The Ds now prevail in the academic literature on the effect of the built environment on travel behavior. I do not consider this a good thing.
At a minimum, the terminology is confusing. “Diversity” means the mix of land uses in a given area, but one could easily mistake it to mean the socio-demographic mix of residents of the area, which falls instead under “demographics.” “Design” is usually measured as the connectivity of the street network, not as the aesthetic qualities of the street environment that the word generally implies. The Ds are a catchy shorthand, but not an especially clear one.
Certain values of the D characteristics do often go together, reflecting the era when a particular neighborhood came into being. For example, pre-World War II neighborhoods are more likely to have both grid street networks (“design”) and neighborhood-scale shopping within walking distance (“diversity”). The Ds also tend to go together because they influence each other. Density in particular has a strong effect on other D characteristics: Higher densities support better transit service (“distance to transit”) and a closer proximity of retail and services (“diversity”). Greater land use “diversity,” coupled with better street connectivity (“design”), produces shorter distances to destinations and thus better “destination accessibility.”
Treating the Ds as independent of each other creates the risk of overestimating their influence. It also creates the risk of underestimating their influence. If two or more characteristics together have synergistic effects, they produce a total effect greater than the sum of each independent effect.
Rarely do researchers discuss these relationships, let alone account for them in their analyses. Particularly troubling is the use of density as a predictor of travel behavior without an explanation of how density influences behavior. Density influences the other D characteristics that more directly determine the choices available to travelers and thus more directly shape their travel choices. Researchers too often justify their reliance on density and the other Ds by citing other researchers’ reliance on the same measures. Even a minimal attempt to justify the Ds based on behavioral theory rather than accepted practice would be an improvement.
The concept of accessibility provides a perfect way to [use the built environment’s features that matter]. As usually defined, the level of accessibility from a given place reflects the distribution of destinations around it, the ease with which those destinations can be reached by various modes, and the amount and character of
activity found there. It tells us something about the choices that the built environment offers to travelers.
Studies show strong connections between accessibility and travel behavior. Accessibility is not only a better measure from a research standpoint. It is also a better measure from a practice standpoint. What matters to people is how easy it is for them to get to where they need to be, and how easy it is to access the services they need or want.
Cities promote density to increase accessibility. Using accessibility as the performance measure by which we assess current conditions and proposed policies could shift the public debate away from the scary idea of density, which often provokes hostile responses. Accessibility may not be as catchy as the Ds, but it makes more sense for researchers, practitioners, and the public alike. It is time to be done with the Ds and get back to A.
Research has frequently found significant associations between the selection of walking as a preferred travel mode and aspects of the built environment that influence accessibility such as proximity and variety of destinations (mixed land use), residential density, street connectivity, the existence of sidewalks and grade separation from traffic, neighborhood safety and neighborhood type (Saelens & Handy, 2008). Clifton & Dill, (2005) showed a positive relationship between residential density, better transit services, mixed land use, and the presence of open space with the number of walking trips. However, the choice of walking and cycling is not only influenced by the built environment; it can also be affected by other factors. For example, Cao, et al., (2006) highlighted that walking frequency is linked to perceived neighborhood safety, traffic conditions, proximity to local destinations, quality of local opportunities, and comfort levels. In short, as shown in Figure 5.4, there are four general categories of factors (built environment, natural environment, social, and personal) that affect walking and cycling activity levels. It is worth noting that accessibility variables of the built environment such as access to opportunities and land-use patterns exert a more pronounced influence on walking and cycling as a mode of transport (Handy et al., 2002; Saelens & Handy, 2008).

Built Environment
The shortest distance or travel time to a destination made possible by the available street network is the primary factor for either modality. This can also be influenced by neighborhood design and location and design of walking and biking paths.
Safety from traffic exposure (traffic volume, speed, and proximity) and safety of the designated paths is the second most crucial factor. Pedestrians are concerned about the walkways and frequent safe crossings in areas with high vehicular traffic volumes and speed. Pedestrians are also averse to traveling near speeding vehicles when walking along a busy street or highway (Kuzmyak et al., 2014) and in not well-lit places at night.
Natural Environment
Factors related to the natural environment include climate, temperature extremes, precipitation, hours of daylight or darkness, and topography. McGinn et al. (2007) examined perceived measures of the natural environment to understand its relationship with physical activity. For instance, they discovered that weather acts as a barrier to physical activities like walking and biking. Moreover, the presence of hills can reduce the likelihood of walking. Conversely, residents of neighborhoods with more tree-shaded streets are more inclined to walk (McGinn et al., 2007; Schiller & Kenworthy, 2010) (Figure 5.2).
Social Environment
Perceived or real neighborhood safety (e.g., crime, vandalism, racial discrimination, neighborhood decay) influences active modes of transportation.
Studies of older adults (age> 65) confirm this. Cerin et al. (2017) meta-analysis of 42 studies of walking and biking activity among elderly populations found positive associations between built environment (from residential density and street connectivity to pedestrian-friendly features, and access to specific destinations), and negative correlations with social environment factors (littering, vandalism, and neighborhood decay).
Personal, Sociodemographic Differences:
Age, gender, race and ethnicity, socioeconomic status, health status, and physical ability.
Cultural attitudes and comfort level or tolerance to stress levels associated with any of the above barriers to walking or biking.
Gender, age, income, vehicle ownership, education, and ethnicity are sociodemographic characteristics that influence people’s decisions to walk or bike. For example, in a study by Saelens et al. (2003) analyzing five major urban areas in the US, it was found that factors such as car ownership, age, possession of a driver’s license, employment status, and household size are negatively correlated with the likelihood of walking and cycling. Conversely, having a professional job and being male were found to have a positive impact on the choice of walking and cycling.
Age and physical ability are significant factors to consider. In many car-oriented suburban areas, walking is often limited to exercise or leisure due to the lack of access to suitable destinations, making it impractical for transportation purposes. While cycling offers more potential destinations compared to walking, both modes require considerations of directness and safety. Kuzmyak et al. (2014) suggest that only individuals who are experienced and determined are likely to commute by bicycle in such conditions (refer to Table 5.2).
Mode | Attitudes and perceptions |
---|---|
Walking | Primary reasons for not walking: Health or disability (24.5%), weather (22%), too busy (18.8%) (National Survey of Bicycle and Pedestrian Attitudes & Behavior, 2002)
Minor reasons for not walking: Other transportation is faster (4%), do not like to walk (3.5%), own a vehicle, and prefer to drive (2.5%) (National Survey of Bicycle and Pedestrian Attitudes & Behavior, 2002). Safety: The presence of traffic control devices and safe vehicle speeds ranked 2nd and 3rd after the shortest distance (Weinstein and Schinek, 2005). Security: The elderly, minorities, and women are most likely to curtail walking travel due to concerns about personal safety after dark (Committee on Physical Activity, health, and Transportation, 2005). |
Bicycling | Safety appears to be the overriding factor influencing attitudes toward and willingness to travel by bicycle; All riders are apprehensive about riding in motor vehicle traffic and will deviate from the shortest route to avoid streets with heavy traffic; regular/experienced riders may be less concerned about traffic safety than infrequent/inexperience riders, but they still demonstrate a preference for routes/ facilities that buffer them from traffic (Dill & Gliebe, 2008; Hunt & Abraham, 2006; Krizek/NCHRP 552; Sener & Bhat, 2010). |
Note: Adapted from “Walking and Bicycling in the United States: The Who, What, Where, and Why,” by Kuzmyak, et al., 2014 (https://onlinepubs.trb.org/onlinepubs/trnews/trnews280www.pdf). |
TRANSIT MODES
Public transportation encompasses various modes such as buses, light rail, subways, commuter trains, streetcars, cable cars, vanpool services, ferries, and water taxis. These transit modes are further discussed in Chapter 6, dedicated to transit. This section provides a short overview of transit modes’ trends and current usage.
Public transit modes are generally accessible to the public for a fare and operate on scheduled times. They aim to enhance access to local and regional destinations and contribute to reducing traffic congestion and vehicle miles traveled (VMT) by automobiles. Public transit systems can be either local or regional and often rely on a mix of federal, state, regional, and local government funding initiatives.
Regional bus networks, including express buses or bus rapid transit (BRT) services, along with specialized for-hire services like airport and train station shuttles, as well as regional rapid transit (RRT) or subway metro systems, all play essential roles in facilitating commuter and traveler movement to and from regional centers. Additionally, regional rail networks, sometimes erroneously referred to as commuter rail, offer another viable transportation option. In certain areas, ferries and water taxis are essential for transporting people to and from cities.

Despite increases in transit ridership worldwide, the United States has experienced consistently low ridership, with a sharp decline observed from 2020 to 2021 which has only partially rebounded, as depicted in figure 5.5. According to data from the Federal Transit Administration, public transit ridership decreased by a greater percentage from 2014-2019 than 2009-2014 in most major markets. Notable exceptions include Atlanta, where the percentage decrease was greater in 2009-2014 than 2014-2019 and Seattle where ridership has not experienced a decrease, just a reduction of the increase. (Figure 5.5 & 5.6).
Erhardt et al. (2022) conducted a study to uncover the factors contributing to this decline between 2012 and 2018. Their findings revealed that lower gas prices, higher transit fares, changes in household income, and the rise of remote work all played a role in the decrease in transit ridership. However, the emergence of on-demand and ride-hailing services offered by Transportation Network Companies (TNCs) such as Uber and Lyft were also identified as the primary driver behind the decline, particularly impacting bus and rail ridership in medium-sized metropolitan areas and bus ridership in large metros. This shift can be attributed to the demand for door-to-door short trips in city centers, where ride-hailing services outcompete traditional bus services. Rail trips, on the other hand, remain popular due to their longer distances and time-saving benefits by avoiding congestion, especially in larger cities. In summary, the study concluded that ride-hailing services significantly impact bus ridership, followed by factors such as gas prices, fares, and the socio-economic characteristics of transit riders.
The structure of a transit system’s services, such as whether bus lines converge on a city center or are distributed throughout a city or region, along with the land use patterns of its service area, including built environment characteristics, density, and centrality, influence bus ridership. Conversely, changes in gas prices and rail transit fares, as well as income and household characteristics, primarily affect rail ridership. Research indicates that service structure has the most significant positive impact on bus ridership, followed by land use patterns (Erhardt et al., 2022). As discussed previously the Covid-19 pandemic further impacted transit in all major transit markets.

INTERMODAL TRANSPORTATION
Passenger intermodality
As discussed earlier, passenger intermodalism involves using multiple modes of transportation for a single journey, a practice commonly known as mixed-mode commuting when applied to daily travel. This approach relies on coordination and integration between different transportation modes, often facilitated by transport agencies and mobile apps, and may necessitate intermodal facilities like terminals for seamless passenger transfers. Achieving a seamless journey with multiple modes requires integrating various services and components, such as information, ticketing, infrastructure, and hubs. Ultimately, when different transportation services are effectively connected and integrated, intermodal mobility can be realized on both urban and regional scales. Figure 5.7 displays the extent of US passenger intermodal facilities by mode in 2022. Of the close to 15,500 intercity and transit rail, air, intercity bus, ferry, and bike-share stations in the US, over one-half (61%) provide an intermodal connection to other public passenger transportation modes. After bikeshare stations, heavy rail transit is the most intermodally connected with 89% of all its 1,043 facilities offering connections to other modes, followed by commuter rail (71% of 1,167 facilities) and light rail (67% of 1,554 facilities) (USDOT 2023, p. 1-37).

An example of a regional intermodal terminal is downtown San Francisco’s Transbay Transit Center (a.k.a Salesforce Transit Center) administered by the Transbay Joint Powers Authority (TJPA). It currently serves as a bus terminal for several lines including AC transit, Greyhound lines, several Muni bus and trolley lines operated by the San Francisco Municipal Transit, WestCAT-Lynx, and Golden Gate Transit. Future phases of this intermodal hub will include a rail terminal to be served by Caltrain and the California High-Speed Rail (see Figures 5.8 through 5.10).

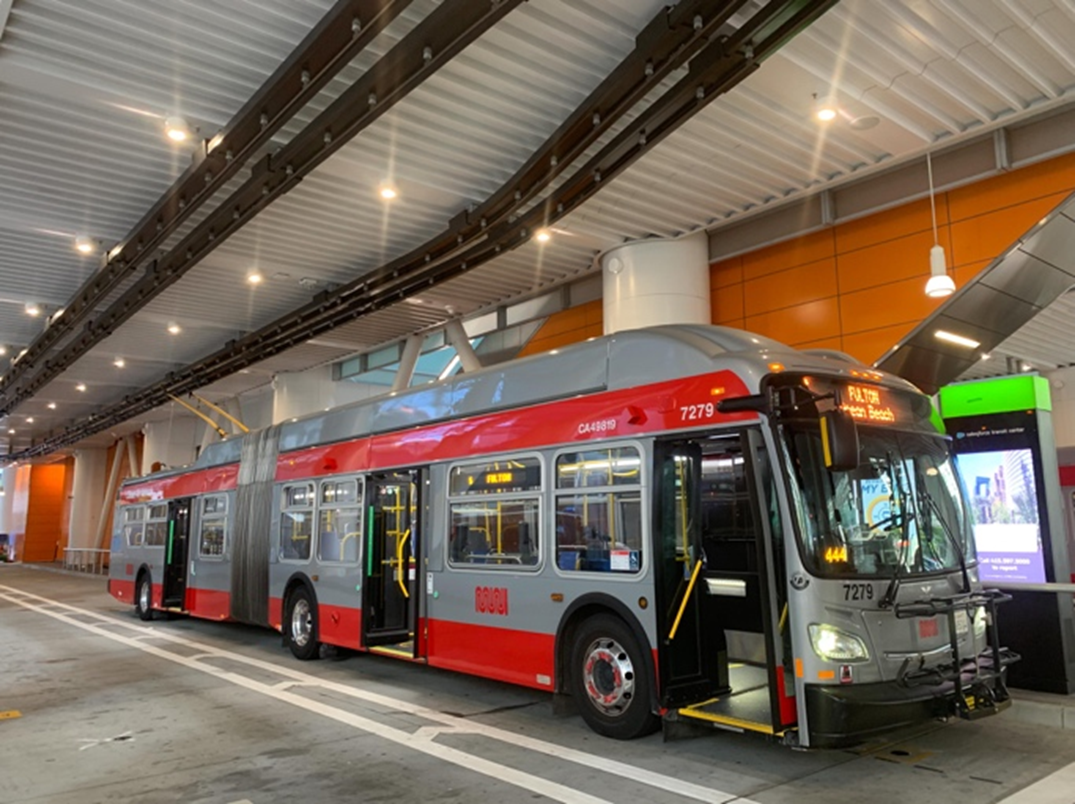

By combining different modes and providers of travel for a single trip, intermodality can help reduce reliance on private vehicles and enable cities to develop more efficient and sustainable mobility systems (Oostendorp & Gebhardt, 2018). This goal is exemplified by the seamless interchanges between different modes in major European cities. For instance, in Berlin, intermodal trips involving bicycles and public transit are prevalent in the urban core, while combining cars with public transit is more common in decentralized neighborhoods. In well-connected areas, a mix of all modes is often observed. From the user perspective, time efficiency and seamless interchanges between modes are prime considerations in choosing intermodal travel for commuting to work (Oostendorp & Gebhardt, 2018).
A word on multimodal mobility
As already mentioned, multimodality refers to the presence of diverse transportation modes, encompassing public transit like buses and trains, waterways, bike networks, pedestrian pathways, and driving. It emphasizes accommodating the needs of all users by providing multiple modes for different purposes. In contrast, intermodality focuses on facilitating seamless connections between these different transportation modes.
An illustration of multimodal transportation planning is the concept of complete streets, which designate thoroughfares that allocate space not only for cars but also for alternative modes like biking, walking, and transit. This approach ensures that all types of travelers can navigate freely without encountering safety or accessibility issues. For more information on complete streets, visit the Federal Highway Administration’s “Complete Streets” at https://highways.dot.gov/complete-streets The reader is also encouraged to visit the OER Multimodal transportation planning by Chen, Boyd, and Williams (2024) at https://uta.pressbooks.pub/oertransportmultimodalplanning/
Given that transportation equity primarily concerns meeting the mobility needs of all demographic groups, including those who may lack access to personal vehicles, the development of multimodal transportation aligns closely with transportation equity objectives. By offering a range of transportation options, multimodal systems facilitate convenient movement for people from diverse backgrounds and circumstances (Litman, 2013).
Freight Intermodality
Intermodal transportation extends beyond passenger travel and encompasses the movement of goods using at least two modes, such as trucks and rail. Typically, these mode transfers occur at intermodal terminals. In the realm of freight transport, intermodal operations play a significant role in international commerce, often involving a multimodal chain of container-transportation services. This chain links an origin (shipper) with a distant destination (consignee) through what’s known as door-to-door service, all integrated under a single ticket (Crainic & Kim, 2007). These intermodal networks can span various carriers, modes (road, rail, water, air), and terminals, operating at regional, international, and intercontinental levels.
A classic example of an intercontinental chain starts with cargo containers transported by truck to a rail yard, often referred to as inland port facilities or terminals. From there, they are transferred to a seaport terminal for shipment across the ocean via ships. Upon arrival at another port, the containers are then transported by land (truck or rail) through different inland port terminals to reach their final destination.
An illustration of an inland port intermodal terminal is the Dallas Intermodal Terminal (DIT), managed by the Union Pacific rail. Situated 12 miles south of downtown Dallas within the cities of Hutchins and Wilmer in Dallas County, this expansive 360-acre facility incorporates advanced technology for efficient operations. Through a state-of-the-art, biometric-secured automated gate system, trucks can swiftly access the airport. This technology enables truckers to process a container through the gate in 30-90 seconds, significantly quicker than the national average of four minutes (DIT, 2015, p.1). This facility is part of the International Inland Port of Dallas (IIPOD), an intermodal and logistics district spanning five municipalities and connecting local, regional, and global markets. The IIPOD leverages the DFW area’s strategic location in North Texas situated at the crossroads of the NAFTA rail and highway corridors which makes the region one of the most strategically advantageous freight logistics hubs in the nation. This geographic significance is exemplified by Alliance, Texas, a sprawling 27,000-acre logistics district positioned north of Fort Worth. Alliance, Texas features a diverse array of truck, rail, and air intermodal facilities and is home to major entities such as BNSF Railways, Amazon, FedEx, USPS, Walmart, and Volkswagen, alongside numerous warehousing, transshipment, and distribution hubs. These facilities connect to major seaports like Los Angeles and Long Beach, as well as cargo airports (Figures 5.10 and 5.11).


Modal choice in the freight industry is a function of transport costs and distance. Truck transportation is most suitable for short distances below 500 km (310 miles), rail for distances between 500 to 750 km (310 to 566 miles) and maritime shipping for the longest distances above 750 km. Intermodal systems are typically networks of sophisticated intermodal chains whose logistics integrators continuously strive to minimize time and costs while enhancing the system’s capacity to efficiently route freight and reduce transshipment durations.
Intermodality and Containerization
The adoption and standardization of containers in the freight industry, facilitated by ISO standards, have played a pivotal role in enabling global commerce and intermodal transportation of goods. Containerization revolutionized the industry by allowing for economies of scale, particularly for non-bulk commodities, and by establishing a new container-based intermodal transportation system with specialized terminals, where the container serves as the fundamental unit. Consequently, the container has become integral to production, transportation, and distribution processes. This shift led to the obsolescence of older ports and prompted the establishment of new sea and inland ports in peripheral locations.
The advent of containerization fueled a significant surge in global trade growth since the 1990s, a trend that has continued to escalate. However, the expansion of intermodal transportation, particularly in the realm of international trade, has exposed inherent transactional costs and inefficiencies. To address these challenges, innovations such as blockchain technology and distributed electronic ledgers have emerged. These technologies aim to support the intricate array of transactions and information flows associated with intermodal transportation, streamlining processes and enhancing efficiency (Rodrigue, 2020a).
Smart Mobility and Enabling Technologies
The convergence of technologies such as the Internet of Things (IoT), electric and autonomous vehicles (EVs and AVs), geolocation (GPS), artificial intelligence (AI), and the widespread use of mobile technology (smartphones) has paved the way for the emergence of transportation mode integrators catering to both people and goods. This technological revolution, often referred to as the 4th industrial revolution, has spurred the development of smart cities worldwide, from New York to San Diego and from Rio de Janeiro to Barcelona, London and Paris. These cities are actively working to enhance their intelligent mobility infrastructures and systems to achieve a more sustainable, equitable, and efficient transportation ecosystem.
Public-private partnerships between cities, transportation authorities, telecom companies, IT mobility startups, car manufacturers, and new transportation network companies (TNCs) are constantly evolving, shaping the public and private technological ecosystem of smart mobility. Mobility as a Service (MaaS) has emerged as a leading innovation aimed at seamlessly integrating various modes of transportation, including public transit, ride-hailing, car and bike-sharing, and other mobility services. While individual solutions like public transportation smart cards, electronic toll cards, and bike-sharing have existed for some time, the concept of smart mobility entails a holistic approach to MaaS. It involves a comprehensive digital platform that offers a seamless travel experience, summarized by Enoch and Potter (2023, n.p.) as including:
- An intermodal journey planner that provides real-time combinations of different transport modes such as car-sharing, car rental, metro, rail, bus, bike-sharing, and taxi.
- A single payment portal allowing users to pay-as-they-go or purchase a pre-arranged service bundle.
- A booking system that encompasses every stage of the entire end-to-end journey.
This integrated approach to smart mobility aims to simplify the travel experience, enhance accessibility, and promote sustainable transportation choices capable of reducing car usage. Over the years, it has become clear that realizing this goal requires to compete with car ownership, a tall order for its implementation. Some analysts have argued that reducing car usage should not be MaaS’s main objective, but rather a complementary measure to enhance public transportation (Orozco-Fontalvo and Moura, 2023).
Indeed, very few city partnerships have successfully achieved the above conditions for integrating available transportation modes. There have been challenges in the mobility tech sector. Instances include bankruptcies, such as MaaS Global’s Whim from Helsinki, Finland (Smith, 2024), and program cancellations, such as X’s Zipster from Singapore (Hammond, 2023), due to limited return on investment for shareholders. Despite these setbacks, several pilot programs in the US have been initiated by cities, transit authorities, and micro-transit tech companies. These initiatives aim to provide on-demand rides in low-density areas with no transit service (transit deserts), like Arlington, TX, or in cities served by buses, such as in King County, WA, New Jersey City, NJ, and Bakersfield, CA see Figure 5.12. The goal in the last cities is to recover lost ridership and stimulate growth after the decline induced by the pandemic.
These programs involve partnerships with mobility tech companies like Via and Remix, which offer integrated intermodal solutions for fixed-route transit (bus and rail), on-demand transit for first-mile and last-mile coverage, transit services in transit deserts, and paratransit community transportation. The video clip shows a session organized by Via featuring the mayors of Arlington and New Jersey City sharing their cities’ experiences with these integrated mobility services at the 2021 US Conference of Mayors.
![]() |
![]() |
Media 5.1 Mayors from Arlington, TX and New Jersey City, NJ talk about their experience with mobility integrated services the US Conference of Mayors. Via (2021, April 14). Via and the US Conference of Mayors host Williams and Fulop for a discussion on the future of transportation [Video]. Vimeo. https://vimeo.com/536777813
Media 5.2 City of Arlington’s AV experience. City of Arlington, TX (2021, August 12). “On the Clock” and On the Road with May Mobility, Arlington’s Autonomous Downtown Shuttle Operator [Video]. YouTube. https://www.youtube.com/watch?v=lYwEp-_hBbw
Media 5.3 Nate Seeskin interviews Principal Planner at the City of Arlington, Texas’ Office of Strategic Initiatives Ann Foss about the city’s Rideshare, Automation, and Payment Integration Demonstration program (or ‘RAPID’ for short) that integrates autonomous vehicles into an existing on-demand ride hail service provided by the city. Shared-Use Mobility Center (2021, November 17). SUMC interview with Ann Foss on Arlington’s RAPID program [Video]. YouTube https://youtu.be/6n6CF_7AgNI
The Future of Smart Mobility
While this section offers a concise glimpse into the future of intermodal transportation, Chapter 9 in this textbook delves deeper into technology and transportation, providing a more comprehensive exploration of the subject.
The future of smart mobility in intermodal transportation is likely to evolve and undergo significant changes driven by technological innovations, policy changes and unfolding transportation trends in several areas: Integration of mobility services: We can expect to see greater integration of more mobility modes beyond public transit and on-demand micro-transit with autonomous vehicles and other micro-mobility modes such as e-bike and scooter sharing and battery-powered slow-speed vehicles for first mile/last mile trips being combined into seamless intermodal transportation networks. Smart mobility platforms will enable passengers to plan, book, and pay for more intermodal trips using a single app, coordinating different modes of transportation. However, challenges related to equity, affordability and time savings for all riders will persist, posing concerns for the business models of mobility integrator companies and their stakeholders, as well as the durability of smart mobility public-private partnerships.
- Data-driven mobility planning: The proliferation of sensors, IoT devices, and connected vehicles and the vast amounts of data generated and harvested through AI and machine learning algorithms will serve to optimize intermodal transportation systems. Analyses of this data will facilitate further real-time insights into traffic patterns, demand fluctuations, and infrastructure conditions, enabling transportation planners to make data-driven decisions and improve the efficiency of passenger and freight movements. However, it also raises concerns regarding data security and privacy, as well as the potential emergence of monopolistic dominant players in the smart mobility industry, presenting future policy and regulatory challenges.
- Electric vehicles (EVs) and smart infrastructure: The shift towards electric modes will continue driven by environmental concerns and regulatory mandates. This shift will likely play a role in reducing carbon emissions and transitioning towards greener intermodal transportation solutions.
- Smart infrastructure and last-mile delivery solutions: investments including connected roadways, smart traffic signals, and intelligent freight terminals, will potentially enhance the efficiency and safety of intermodal freight transportation operations, while the growth of e-commerce will likely promote smart mobility solutions like delivery drones, autonomous robots, and crowd-shipping platforms transforming the last-mile delivery process.
The future of smart mobility in intermodal transportation holds promise for transformative changes driven by technological innovations and evolving transportation trends. The integration of mobility services, enabled by public and private partnerships and driven by tech mobility companies, is beginning to create transportation networks that complement public transportation, particularly at the first-mile and last-mile segments of the travel journey. However, challenges related to equity, affordability, time savings, data security and privacy remain significant issues for smart mobility intermodal initiatives.
CONCLUSION
Understanding travel modes reveals their multifaceted role in transportation planning and policy. A well-planned transportation system effectively supports and safely accommodates all modes of transportation for both people and goods. By exploring the complexities of transportation modes for people, we gain valuable insights into travel behavior, current and future transportation needs, and opportunities for promoting sustainable alternatives. The importance of active transportation modes, such as walking, cycling, and public transit, cannot be overstated, especially in addressing congestion, reducing air pollution, and fostering healthier, more equitable communities.
However, it’s essential to recognize that each passenger and freight mode is embedded within a broader sociotechnical context or transportation regime. Census data on commuting modal share nationally and from American metropolitan areas highlights the dominance of the car as the primary mode. Moreover, the Covid-19 pandemic significantly increased the share of remote working, leading to declines in the use of other modes, notably public transit—a trend predating the pandemic that persists to this day. Understanding mode share underscores the diverse factors influencing the demand for mobility modes. Research on mode choice has emphasized the design of the built environment as a factor often captured in the 3 D’s (density, diversity, design) and 5 D’s (density, diversity, design, destination accessibility, and distance) studies. However, this perspective has been challenged by other researchers underscoring “A” for accessibility over “D” for design. Additionally, active transportation research has explored social, personal, and environmental factors beyond just the built environment.
Passenger intermodality emphasizes the importance of seamlessly connecting different transportation modes to enhance travel experiences. This requires collaboration among transport agencies, mobile app developers, and infrastructure planners to integrate services such as information, ticketing, and facilities. By doing so, mobility options can be improved on both urban and regional scales. In freight intermodality, the integration of various modes, such as trucks, rail and ships, is essential for optimizing logistics chains and improving efficiency in international commerce. Containerization, as a cornerstone of intermodal freight transport, has revolutionized supply chain management by enabling economies of scale and streamlining transportation processes through specialized ports and terminals. The advent of smart mobility technologies, driven by the ongoing IT revolution, presents new opportunities for enhancing intermodal transportation systems. Smart cities worldwide are leveraging public-private partnerships to develop intelligent mobility infrastructures and systems aimed at achieving sustainability, equity, and efficiency in transportation. Although Mobility as a Service (MaaS) holds promise for seamlessly integrating various transportation modes, its full implementation has yet to materialize. Filling this gap, demand response microtransit companies in the US have begun to serve transit-deserts and as transit feeders to meet the mobility needs of low-density areas. While the future of smart mobility in intermodal transportation holds promise for transformative change, challenges such as equity, affordability, data security, and privacy must be addressed to ensure inclusive and sustainable mobility solutions.
Car-oriented development patterns refer to urban areas designed with a primary focus on accommodating cars over other modes of transportation, thereby making car travel the predominant mode. These patterns are characterized by large city blocks, wide streets, and extensive parking areas. Car-centric development has been widespread in the US since the 1960s.
Commuting refers to travel between home and work.
Complete streets are part of a transportation planning strategy that ensures the transportation network is designed and maintained to accommodate safe mobility for all modes and road users, including pedestrians, bicyclists, motorists, and transit riders of various ages and abilities (McCann et al, 2023).
Containers applied to freight are a standardized metal box used for shipping goods domestically or internationally by sea, rail, or road.
Demand response (DR) or On-Demand refers to a transit mode comprised of passenger cars, vans or small buses operating in response to calls from passengers or their agents to the transit operator, who then dispatches a vehicle to pick up the passengers and transport them to their destinations (FTA, 2024).
Euclidean distance is the length of the line segment that connects two points.
First-mile and last-mile refers to the initial and final segments of a journey, typically between a transportation hub (such as a train station or bus stop) and the traveler’s origin or destination.
Geolocation refers to the process of determining and identifying the geographical location of a device or a person using various technologies such as GPS, Wi-Fi, or cellular networks.
Intermodal facilities are terminals linking at least two transportation modes. In passenger transportation, airports serve as intermodal facilities connecting land and air modes. For freight, they can be port terminals connecting water and land modes (such as ships, barges, and rail); rail terminals linking other land modes (rail and trucks); and truck-based distribution centers and warehousing hubs connecting land modes (trucks and rail) and water modes (ships or barges).
Internet of things refers to a network of interconnected devices (from smart phones, computers and appliance to car transponders, security cameras and cities) and objects like sensors that can communicate and exchange data over the internet without human intervention.
ISO standards are internationally recognized guidelines or specifications established by the International Organization for Standardization to ensure quality, safety, and efficiency in various industries and processes.
Logistics in freight transportation refers to container-based intermodal transportation of bulk goods for the long haul. Carriers use different freight modes and intermodal facilities to move cargo to its final destination.
Micro-mobility modes refer to small, lightweight, and usually electric vehicles or devices used for short-distance urban transportation, such as electric scooters, bicycles, and electric skateboards.
Mobility as a service (MaaS) is a transportation concept that integrates various modes of transportation into a single service accessible through a digital platform, allowing users to plan, book, and pay and seamlessly experience their journey.
Network-based distance is the realistic distance between an origin and a destination that a pedestrian or traveler would traverse over a street network to reach the destination.
Paratransit in the US is an individualized form of public transportation service without fixed routes and timetables. It is often designed to meet the mobility needs of individuals with disabilities or those who are unable to use conventional public transportation.
Public Transportation As defined in the Federal Transit Act, “transportation by a conveyance that provides regular and continuing general or special transportation to the public” (FTA, 2024).
Smart cities are urban areas that utilize technology—the IoT, and data-driven solutions to improve efficiency of operations, sustainability, and quality of life for its residents.
Transit desert refers to an area with limited or no access to public transportation services, making it difficult for residents without private cars to travel affordably within the area or to other destinations.
Transit feeder is a transportation service that connects passengers from an origin point to a main transit system (rail or bus on main transportation routes).
Transportation network companies (TNC) also known as ride-hailing or rideshare companies, utilize mobile phone apps to offer on-demand transportation and delivery services.
Transportation mode: A transportation mode refers to the specific method used for traveling or transporting people and goods, each relying on distinct infrastructure, vehicles, operators, and operations. Except for walking, modes rely on specific mobility technologies and fuel for movement on land, air, or water.
Transportation regime: In the socio-technical transitions perspective, a regime encompasses the social, material, and technological elements that maintain and reproduce a socio-technical system. These regimes encompass various dimensions, including technology, government regulations, infrastructure, markets, cultural values, and social practices. Each travel mode within the road passenger transportation system is conceptualized as a regime, with the car being the dominant regime, while modes like public transit or cycling are secondary in comparison.
Unlinked Passenger Trips (UPT) are the number of passengers who board public transportation vehicles. Passengers are counted each time they board vehicles no matter how many vehicles they use to travel from their origin to their destination (FTA, 2024).
REFERENCES
Brunner, H., Hirz, M., Hirschberg, W., & Fallast, K. (2018). Evaluation of various means of transport for urban areas. Energy, Sustainability and Society, 8(1), 9. https://doi.org/10.1186/s13705-018-0149-0
Cao, X., Handy, S. L., & Mokhtarian, P. L. (2006). The influences of the built environment and residential self-selection on pedestrian behavior: Evidence from Austin, TX. Transportation, 33, 1–20.
Cerin, E., Nathan, A., van Cauwenberg, J., Barnett, D. W., Barnett, A., & Council on Environment and Physical Activity (CEPA) – Older Adults working group (2017). The neighborhood physical environment and active travel in older adults: a systematic review and meta-analysis. The international journal of behavioral nutrition and physical activity, 14(1), 15. https://doi.org/10.1186/s12966-017-0471-5
Cervero, R., & Kockelman, K. (1997). Travel demand and the 3Ds: Density, diversity, and design. Transportation Research Part D: Transport and Environment, 2(3), 199–219.
Cervero, R., Rood, T., & Appleyard, B. (1995). Job accessibility as a performance indicator: An analysis of trends and their social policy implications in the San Francisco Bay Area.
Cheng, J., & Bertolini, L. (2013). Measuring urban job accessibility with distance decay, competition and diversity. Journal of Transport Geography, 30, 100–109.
City of Arlington, TX (2021, August 12). “On the Clock” and On the Road with May Mobility, Arlington’s Autonomous Downtown Shuttle Operator [Video]. YouTube. https://www.youtube.com/watch?v=lYwEp-_hBbw
Clifton, K. J., & Dill, J. (2005). Women’s travel behavior and land use: Will new styles of neighborhoods lead to more women walking? Transportation Research Board Conference Proceedings 35. http://onlinepubs.trb.org/onlinepubs/conf/CP35v2.pdf
Crainic, T. G., & Kim, K. H. (2007). Chapter 8 Intermodal Transportation. In C. Barnhart & Laporte, Handbooks in operations research and management science, 14, 467–537. Elsevier. https://doi.org/ 10.1016/S0927-0507(06)14008-6
El-Geneidy, A. M., & Levinson, D. M. (2006). Access to Destinations: Development of Accessibility Measures. http://conservancy.umn.edu/handle/11299/638
Erhardt, G. D., Hoque, J. M., Goyal, V., Berrebi, S., Brakewood, C., & Watkins, K. E. (2022). Why has public transit ridership declined in the United States? Transportation Research Part A: Policy and Practice, 161, 68–87.
Ewing, R., & Cervero, R. (2001). Travel and the built environment: A synthesis. Transportation Research Record, 1780(1), 87–114.
Ewing, R., & Cervero, R. (2010). Travel and the built environment: A meta-analysis. Journal of the American Planning Association, 76(3), 265–294.
Federal Highway Administration. (2017). National household travel survey. Technical Report.
Federal Transit Administration (FTA). (2024). National Transit Database (NTD) Glossary https://www.transit.dot.gov/ntd/national-transit-database-ntd-glossary
Geels, F. W. (2018). Low-carbon transition via system reconfiguration? A socio-technical whole system analysis of passenger mobility in Great Britain (1990–2016). Energy Research & Social Science. 46 (2018), 86-102. https://doi.org/10.1016/j.erss.2018.07.008
Geurs, K. T., & van Eck, J. R. (2003). Evaluation of accessibility impacts of land-use scenarios: The implications of job competition, land-use, and infrastructure developments for the Netherlands. Environment and Planning B, 30(1), 69–88.
Handy, S. L., Boarnet, M. G., Ewing, R., & Killingsworth, R. E. (2002). How the built environment affects physical activity: Views from urban planning. American Journal of Preventive Medicine, 23(2, Supplement 1), 64–73. https://doi.org/10.1016/S0749-3797(02)00475-0
Hansen, W. G. (1959). How accessibility shapes land use. Journal of the American Institute of Planners, 25(2), 73–76.
Hirschhorn, F., Paulsson, A., Sørensen, C.H. and Veeneman, W. (2019). Public transport regimes and mobility as a service: Governance approaches in Amsterdam, Birmingham, and Helsinki. Transp. Res. Part A Policy Pract., 130 (2019), 178-191.
Hoffmann, S. Weyer, J. and Longen, J. (2017). Discontinuation of the automobility regime? An integrated approach to multi-level governance. Transp. Res. Part A Policy Pract., 103 (2017), 391-408.
Intermodal passenger transport. (2022, February 3). In Wikipedia. https://en.wikipedia.org/wiki/Intermodal_passenger_transport
Kuzmyak, J. R., Walters, J., Bradley, M., & Kockelman, K. M. (2014). Estimating bicycling and walking for planning and project development: A guidebook (Issue Project 08-78). https://www.trb.org/Publications/Blurbs/171138.aspx
Lai, Z., Wang, J., Zheng, J., Ding, Y., Wang, C., Zhang, H. (2023). Travel mode choice prediction based on personalized recommendation model. IET Intell. Transp. Syst. 17, 667–677. https://doi.org/10.1049/itr2.12290
Litman, T. (2013). Toward more comprehensive and multi-modal transport evaluation. Victoria Transport Policy Institute.
Lv, Y., Duan, Y., Kang, W., Li, Z., Wang, F.Y.(2014). Traffic flow prediction with big data: a deep learning approach. IEEE Trans. Intell. Transp. Syst. 16(2), 865–873.
Mallett, W. J. (2018). Trends in public transportation ridership: Implications for federal policy (Doctoral dissertation). George Washington University. https://sgp.fas.org/crs/misc/R45144.pdf
McCann, B, Boutros, A, and Biton, A. (2023). Complete streets: Prioritizing safety for all road users. Public Roads, 86 (4), FHWA-HRT-23-002. https://highways.dot.gov/public-roads/winter-2023/complete-streets-prioritizing-safety-all-road-users
McGinn, A. P., Evenson, K. R., Herring, A. H., & Huston, S. L. (2007). The relationship between leisure, walking, and transportation activity with the natural environment. Health & Place, 13(3), 588–602. https://doi.org/10.1016/j.healthplace.2006.07.002
Mode of transport. (2022, June 25). In Wikipedia. https://en.wikipedia.org/w/index.php?title=Mode_of_transport&oldid=1094970100
National Research Council (US). Committee for Determination of the State of the Practice in Metropolitan Area Travel Forecasting. (2007). Metropolitan travel forecasting: Current Practice and future direction—Special report 288 (Issue 288). Transportation Research Board.
Oostendorp, R., & Gebhardt, L. (2018). Combining means of transport as a users’ strategy to optimize traveling in an urban context: Empirical results on intermodal travel behavior from a survey in Berlin. Journal of Transport Geography, 71, 72–83.
Regional Plan Association (RPA). (1929 May). Regional plan of New York and its environs. https://rpa.org/work/reports/regional-plan-of-new-york-and-its-environs
Rodrigue, J.P. (2020). Transportation as a derived demand. The geography of transport systems. Routledge. https://transportgeography.org/contents/chapter1/what-is-transport-geography/transportation-derived-demand/.
Saelens, B. E., & Handy, S. L. (2008). Built environment correlates of walking: A review. Medicine and Science in Sports and Exercise, 40(7 Suppl), S550.
Saelens, B. E., Sallis, J. F., & Frank, L. D. (2003). Environmental correlates of walking and cycling: Findings from the transportation, urban design, and planning literatures. Annals of Behavioral Medicine, 25(2), 80–91.
Sarvepalli, M. (2019, September 7). Role of blockchain in transforming India’s multi-modal transportation. Medium. https://malliksarvepalli.medium.com/role-of-blockchain-in-transforming-indias-multi-modal- transportation-6977580f4ff3
Schiller, P. L., & Kenworthy, J. R. (2010). An introduction to sustainable transportation: Policy, planning, and implementation. Routledge. https://doi.org/10.4324/9781849775021
Schiller, P.L., & Kenworthy, J. (2017). An Introduction to Sustainable Transportation: Policy, Planning and Implementation (2nd ed.). Routledge. https://doi.org/10.4324/9781315644486
Shared-Use Mobility Center (2021, November 17). SUMC interview with Ann Foss on Arlington’s RAPID program [Video]. YouTube https://youtu.be/6n6CF_7AgNI
U.S. DOT (2023). Transportation statistics annual report 2023. Bureau of Transportation Statistics, Washington, DC. https://doi.org/10.21949/1529944
Via (2021, April 14). Via and the US Conference of Mayors host Williams and Fulop for a discussion on the future of transportation [Video]. Vimeo. https://vimeo.com/536777813
Walton, R. O. (2014). The 6th mode of transportation. Journal of Transportation Management, 25(1), 55-61. https://doi.org/10.22237/jotm/1396310700
Wang, Y., Chau, C. K., Ng, W. Y., & Leung, T. M. (2016, February). A review of the effects of physical built environment attributes on enhancing walking and cycling activity levels within residential neighborhoods. Cities, 50, 1–15. https://doi.org/10.1016/j.cities.2015.08.004
Whitfield, G. P. (2020). Monitoring state-level changes in walking, biking, and public transit to work-American Community Survey, 2006 and 2017. Preventing Chronic Disease, 17, https://doi.org/10.5888/pcd17.200097
Transportation mode: A transportation mode refers to the specific method used for traveling or transporting people and goods, each relying on distinct infrastructure, vehicles, operators, and operations. Except for walking, modes rely on specific mobility technologies and fuel for movement on land, air, or water.
Mode: The means used for travel (e.g., walking, driving your car, riding as a passenger on a bus).
Transportation regime: A standardized way or dominant mode of personal mobility, including the technological, industrial, political, and cultural elements of transportation (Hoffmann, Weyer, & Longen, 2017).
Intermodal facilities are terminals linking at least two transportation modes. In passenger transportation, airports serve as intermodal facilities connecting land and air modes. For freight, they can be port terminals connecting water and land modes (such as ships, barges, and rail); rail terminals linking other land modes (rail and trucks); and truck-based distribution centers and warehousing hubs connecting land modes (trucks and rail) and water modes (ships or barges).
Car-oriented development patterns refer to urban areas designed with a primary focus on accommodating cars over other modes of transportation, thereby making car travel the predominant mode. These patterns are characterized by large city blocks, wide streets, and extensive parking areas. Car-centric development has been widespread in the US since the 1960s.
Commuting refers to travel between home and work.
Euclidean distance is the length of the line segment that connects two points.
Network-based distance is the realistic distance between an origin and a destination that a pedestrian or traveler would traverse over a street network to reach the destination.
Public transportation, as defined in the Federal Transit Act, "transportation by a conveyance that provides regular and continuing general or special transportation to the public” (FTA, 2024).
Demand response (DR) or On-Demand refers to a transit mode comprised of passenger cars, vans or small buses operating in response to calls from passengers or their agents to the transit operator, who then dispatches a vehicle to pick up the passengers and transport them to their destinations (FTA, 2024).
Transportation Network Companies (TNCs) link drivers utilizing their vehicles with clients via an internet platform (such as a smartphone app) to provide planned transportation services for payment. (FHWA, 2016)
Complete streets are part of a transportation planning strategy that ensures the transportation network is designed and maintained to accommodate safe mobility for all modes and road users, including pedestrians, bicyclists, motorists, and transit riders of various ages and abilities (McCann et al, 2023).
Logistics in freight transportation refers to container-based intermodal transportation of bulk goods for the long haul. Carriers use different freight modes and intermodal facilities to move cargo to its final destination.
Containers applied to freight are a standardized metal box used for shipping goods domestically or internationally by sea, rail, or road.
ISO standards are internationally recognized guidelines or specifications established by the International Organization for Standardization to ensure quality, safety, and efficiency in various industries and processes.
Internet of things refers to a network of interconnected devices (from smart phones, computers and appliance to car transponders, security cameras and cities) and objects like sensors that can communicate and exchange data over the internet without human intervention.
Geolocation refers to the process of determining and identifying the geographical location of a device or a person using various technologies such as GPS, Wi-Fi, or cellular networks.
Smart Cities: City planning and operations utilizing ICT to collect data to manage and improve efficiencies of services such as transportation, utilities, waste management, and criminal investigations. Connected services collect data from citizens, buildings, and other city service nodes and analyze the trends and flows of resources to predict and provide more efficient services (“Smart city,” 2024).
Mobility as a service (MaaS) is a transportation concept that integrates various modes of transportation into a single service accessible through a digital platform, allowing users to plan, book, and pay and seamlessly experience their journey.
Transit desert refers to an area with limited or no access to public transportation services, making it difficult for residents without private cars to travel affordably within the area or to other destinations.
First-mile and last-mile refers to the initial and final segments of a journey, typically between a transportation hub (such as a train station or bus stop) and the traveler's origin or destination.
Paratransit in the US is an individualized form of public transportation service without fixed routes and timetables. It is often designed to meet the mobility needs of individuals with disabilities or those who are unable to use conventional public transportation.
Transit feeder is a transportation service that connects passengers from an origin point to a main transit system (rail or bus on main transportation routes).