10 Mental Health and Multi-Microbiome Interactions
Mental Health and Multi-Microbiome Interactions
It may seem obvious that localized microbiomes are responsible for diseases related to their respective areas (e.g., IBD and the gut microbiome or AD and the skin microbiome), however, it is a fascinating phenomenon that various microbiomes can affect each other and influence health in different parts of the body. Even more captivating is the link between certain microbiomes and mental health conditions presuming the brain is devoid of microbes.
These links between microbiomes are referred to as axes, and initial connections began with the most well-studied microbiome, the gut. Since many gut-derived microbial-produced molecules and compounds are spread through the bloodstream, associations with various organs are formed, such as the gut-brain axis, gut-skin axis, gut-lung axis, or a combination of multiple; gut-brain-skin axis. There are likely overlapping connections between all microbial components that form the human holobiome, thus teasing apart the exact members and their functions is a dutiful task.
Gut Interaction with Other Microbiomes
As mentioned earlier, the dissemination of molecules and compounds from the gut to the rest of the body creates an interconnected highway affecting most, if not all, parts of the body. Certainly, juxtaposed regions such as the oral cavity and the proximal portions of the GI tract have a relationship between their microbiomes, which it termed the gut-oral axis (GOA). These linked microbiomes have been shown to have immunomodulatory roles in the development of rheumatoid arthritis (RA) and osteoarthritis (OA) under dysbiosis, where the abundance of oral Porphyromonas gingivalis and intestinal Prevotella copri could be responsible (Drago et al., 2019, du Teil Espina et al., 2019). It has also been proposed that dysbiosis of the gut-oral microbiome axis is implicated in cirrhosis of the liver through pathogen invasion, resultant systemic inflammation, and impaired immunity and liver function (Acharya et al., 2017). Even gastrointestinal conditions like IBD and cancer of the colon, liver, and pancreas are linked with both the gut and oral microbiome dysbiosis, further demonstrating this strong interorgan connection to human health (Park et al., 2021).
The gut and the skin microbiomes are also linked via the gut-skin axis (GSA), and as the primary interface to the environment, they have major roles in physiological health (Figure 1). Dysbiosis of the gut-skin microbiome axis has influence on both GI and cutaneous disorders such as IBD, celiac disease, atopic dermatitis, psoriasis, acne, and other dermatologic issues, where each of these diseases can be associated with the ‘partner disease’ (i.e. a disease in one system linked to a disease in another system) (O’Neill et al., 2016, Salem et al., 2018, De Pessemier et al., 2021). Though crosstalk is bidirectional, more research seems to have focused on skin disorders and homeostasis as a result of gut microbiome health, where diet, immunomodulation, intestinal permeability, and metabolite secretion are major contributing factors (De Pessemier et al., 2021). However, there is evidence that Mallassezia restricta, a fungal member of the skin microbiota, is associated with Crohn’s disease and can exacerbate colitis (Limon et al., 2019, Sinha et al., 2021).
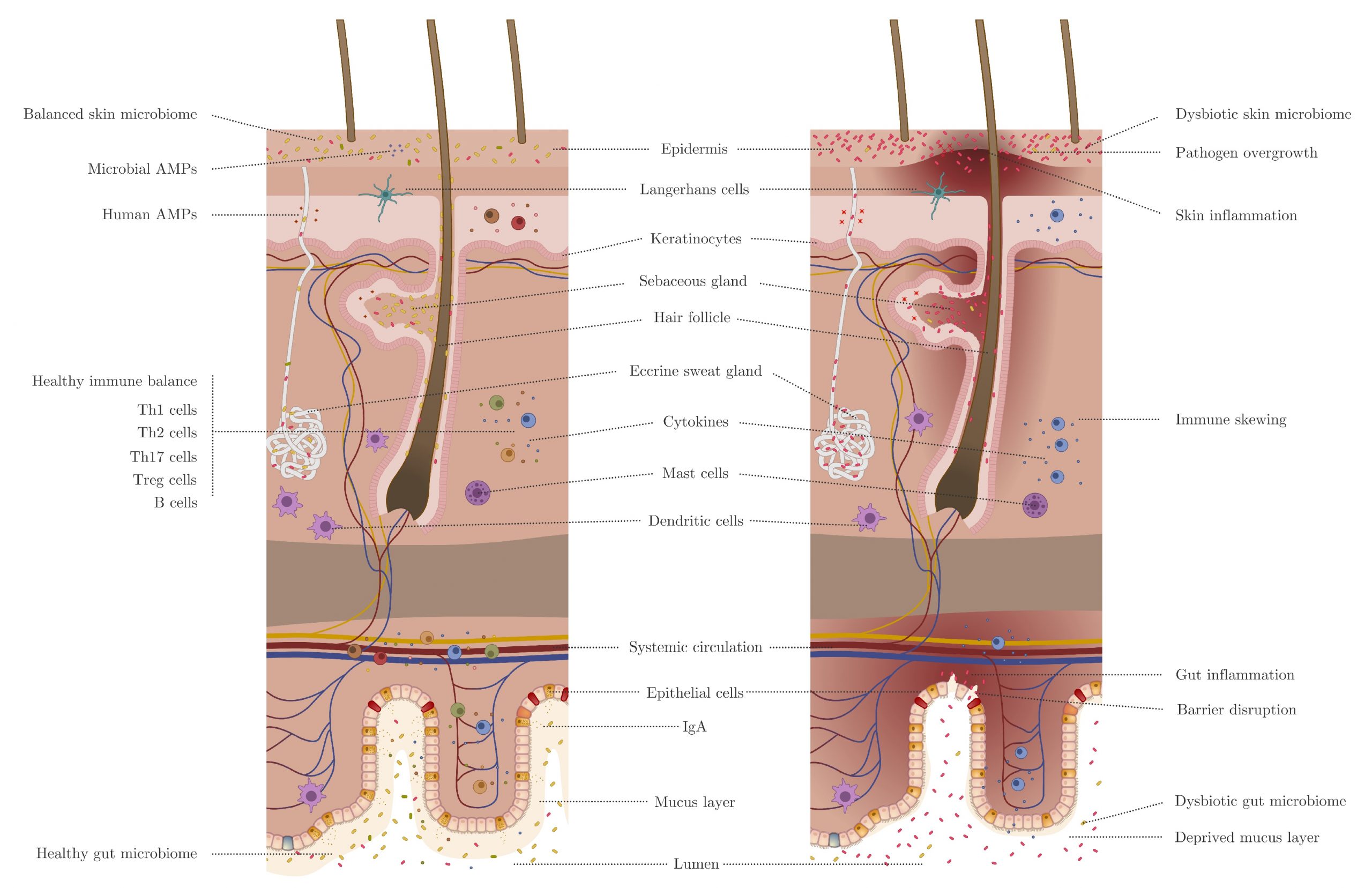
The gut-lung axis (GLA) has roles in the regulation of immunity and the development of various respiratory diseases. (Frati et al., 2019). The respiratory system and GI tract are connected by the mesenteric lymphatic system, where intact or fragmented microbes and their metabolites enter systemic circulation after passing through the intestinal barrier and can migrate to the pulmonary system to modulate immune responses (Enaud et al., 2020). The pathophysiology of diseases such as atopy and asthma are complicated and can be contributed to a variety of factors, however, there is evidence that dysbiosis of the gut microbiome contributes to the development of asthma, particularly in youths who exhibit a decrease in Lachnospira and increase in Clostridium spp. (Penders et al., 2007, Watson et al.,2019). Gut dysbiosis is also involved in chronic obstructive pulmonary disease (COPD) exacerbation, where fiber deficiency in an individual’s diet can contribute to chronic inflammation. Metabolism of fiber by gut microbes produces anti-inflammatory short-chain fatty acids (SCFAs), which could reduce inflammation both systemically and in the lungs, and so targeted dietary intervention for these patients may be a viable treatment addition for COPD and other respiratory diseases associated with inflammation like COVID-19 (Li et al., 2018, Vaughan et al., 2019, Allali et al., 2021). Moreover, SCFAs also have an important role in the defense against secondary infections in those afflicted by viral respiratory infections, further demonstrating the importance of gut microbiome health in connection with the GLA (Sensio et al., 2020).
Like other axes, immunomodulation by the gut microbiome also has influence on the gut-vagina axis (GVA), though it is less extensively studied. One promising avenue of treating vaginal diseases, specifically cervical cancer associated with human papilloma virus (HPV), is the use of mucosal lactic-acid bacteria (LAB)-based vaccines to modulate the gut microbiome. The approach could work as a prophylactic or for direct therapy, and be more easily administered via an oral route instead of parenteral (Taghinezhad-S, et al., 2021). Endometriosis and infertility are also disorders associated with sex hormone levels and inflammation, which once again are influenced by the gut microbiome composition and state. Specifically, there are some members in the gut who can affect the levels of circulating estrogen through metabolic processes and an altered state could lead to increased risk or symptom severity of these disorders (Salliss et al., 2022). Other female reproductive-associated diseases such as polycystic ovarian syndrome (PCOS), also associated with gut dysbiosis, could be ameliorated by diet modification and restoration of gut homeostasis. Here, the use of flaxseed oil could increase diminished levels of SCFAs observed in those with PCOS and protect against inflammation characteristic of the disease (Wang et al., 2020).
The maternal microbiome, including gut, vagina, and breast milk, can greatly influence the colonization and health of the infant after birth, but also affect the fetus prior to delivery. Fetal immune development is most likely influenced in the womb from translocation of maternal gut microbes and/or their metabolites across the placental barrier or ingestion of amniotic fluids (Walker et al., 2017, Nyangahu and Jaspan, 2019). Post-reproduction, the infant gut microbiome is continually developed through initial diet, and more specifically vertical transmission of the contents in the mother’s breast milk which includes its own unique microbiome (Ojo-Okunola et al., 2018, Ojo-Okunola et al., 2019, Quin et al., 2020). The human milk microbiome promotes infant gut colonization of probiotic strains which have roles in programming the immune and metabolic systems, as well as anti-infective, anti-allergic, and anti-tumor properties (Heikkila and Saris, 2003, Olivares et al., 2006, Lara-Villoslada et al., 2007, Civardi et al., 2015, Hassan et al., 2015, Walker and Iyengar, 2015, Boix-Amoros et al., 2016, Ojo-Okunola et al., 2018).
Mental Health Axes
The thought of microorganisms controlling aspects of cognitive function in their host, especially humans, is fascinating to say the least. While connections between other microbiomes, organ systems, and diseases may be less surprising, the microbial link to psychiatric, neurodevelopmental, age-related, and neurodegenerative disorders is very much intriguing. The human microbiome can communicate with the brain in a variety of ways; through the immune system, metabolism, endocrine system, circulatory system, and the nervous system, where microbes, their induced immune response, and their metabolites such as short-chain fatty acids, branched chain amino acids, and peptidoglycans are involved (Figure 2) (Liang et al., 2018, Cryan et al., 2019, Olsen and Hicks, 2019, Hadian et al., 2020, Bear et al., 2021). Several local microbiomes each contribute affects to the mental state, and whether it is gut-, skin-, oral-, lung-, etc. derived can dictate roles in various disorders. This is not just a one-way street though, as microbes around the body can alter mental states but also be affected by governance of the brain in a bi-directional manner (Ma et al., 2019).
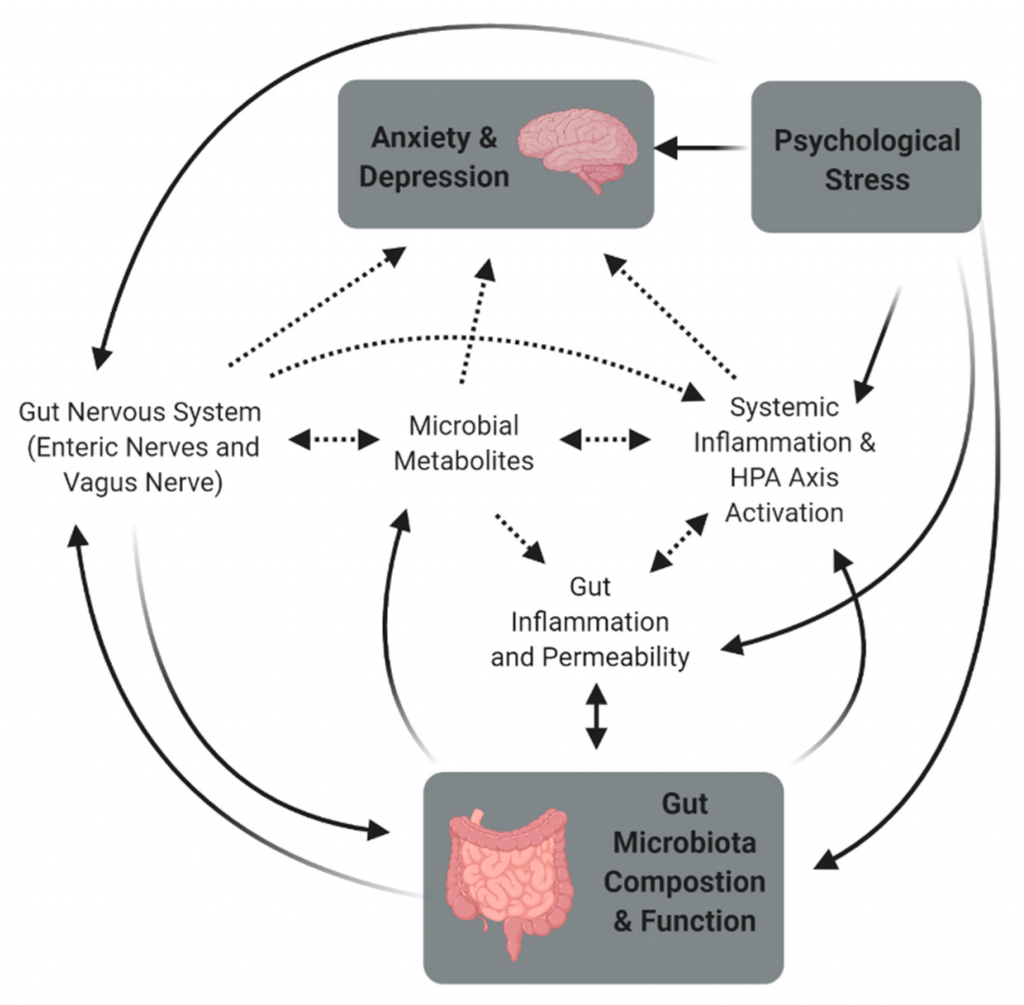
Gut-Brain
Similar to other types of microbiome and organ interactions, the gut’s role in mental health is central and most well studied, which makes sense as it is the largest repository of microorganisms associated with the human body. The gut-brain axis (GBA; and gut plus essentially every other microbiome and brain axis, e.g., gut-skin-brain) is implicated in a number of cognitive functions and disorders including autism, anxiety, depression, stress, pain sensitivity, learning capacities, memory loss, moods and emotions, behavior (dietary, social, and reproductive), schizophrenia, Parkinson’s disease, and Alzheimer’s disease (Desbonnet et al., 2013, Stumpf et al., 2013, Dash et al., 2015, Gareau, 2016, Luczynski et al., 2016, Hoban et al., 2017, Liang et al., 2018, Manderino et al., 2017, Nishida and Ochman, 2017, Vuong et al., 2017, Cowan et al., 2018, Cryan et al., 2019, Bear et al., 2021, Narengaowa et al., 2021). The gut microbiome actually develops in sync with the brain and psychology, and disturbances during different stages of growth can result in the onset of different diseases (Figure 3) (Borre et al., 2014, Gur et al., 2015, Sampson and Mazmanian, 2015, Dinan and Cryan, 2016, Luczynski et al., 2016, Sharon et al., 2016, Kundu et al., 2017, Vuong et al., 2017, Carlson et al., 2018, Liang et al., 2018).
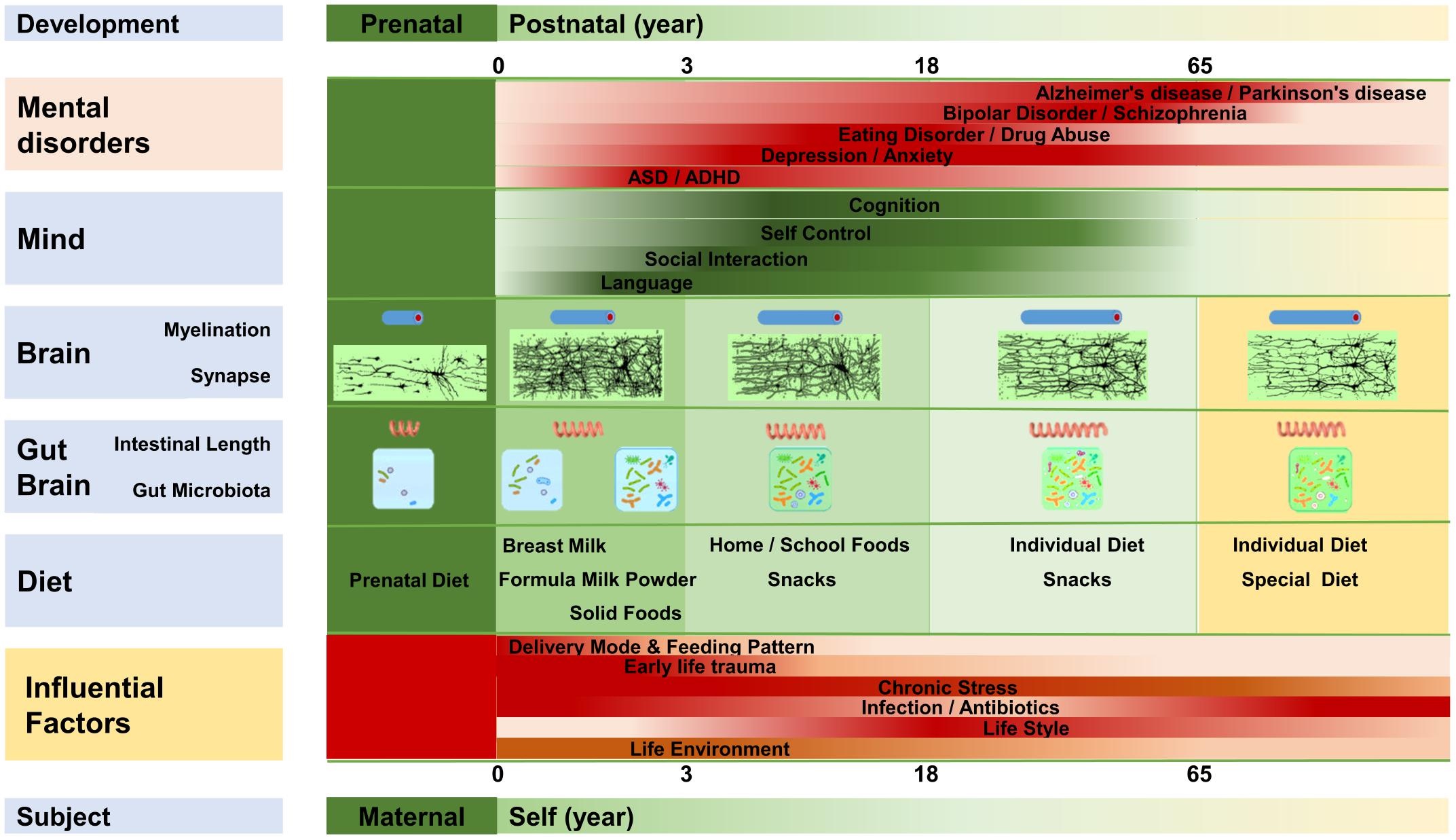
Early perturbation of the gut microbiome during the post-natal period, and even within the womb, can especially increase susceptibility to developing mental disorders since these are critical stages for development of the gut-brain axis and mind (Borre et al., 2014, Gur et al., 2015, Diaz Heijtz, 2016, Mika et al., 2016, Slykerman et al., 2016, O’Mahony et al., 2017, Liang et al., 2018). Even up through senescence, an abnormal gut microbiota is linked with several mental disorders, though the good news is that they can be remedied or improved by returning the gut to a homeostatic state. As the prevalence of mental disorders and neurological diseases have been steadily increasing, healing mental health by exploring and implementing options such as fecal microbiota transplant, diet intervention, probiotics, prebiotics, and psychobiotics are a must (Cryan and Dinan, 2012, Dinan et al., 2013, Liang et al., 2015, Pirbaglou et al., 2016, He et al., 2017, Kang et al., 2017, Mika et al., 2017, Bruce-Keller et al., 2018, Liang et al., 2018, Yang et al., 2018, Kesika et al., 2021, Margolis et al., 2021).
There are several ideas as to why there has been an increase in mental and neurological disorders in relation to the human microbiome. The “Gut Microbiota” hypothesis suggests this escalation is a direct result from gut microbiome dysbiosis due to factors of modern society such as diet, antibiotics, and stress (Liang et al., 2018b). The “Old Friends” hypothesis proposes that the co-evolution of early humanity with its microbiota in a less civilized and more natural ecosystem promoted the development of a stronger immune system, but now changes in lifestyle and environment leads to weaker immunity and subsequent disorders (Strachan, 1989, Rook and Lowry, 2008, Kramer et al., 2013, Rook, 2013). Lastly, the “Leaky Gut” theory implies that damage to the mucosal barrier of the gut increases intestinal permeability, which allows biomolecules and microorganisms to gain access various parts of the body that they normally couldn’t, and thereafter cause disease (Leclercq et al., 2012, Smythies and Smythies, 2014, Kelly et al., 2015, Potgieter et al., 2015, Slyepchenko et al., 2017, Liang et al., 2018). All of theses propositions are clear in their indication that the gut microbiome and its part in immune development has a definite role in the functioning of the mind, though the exact extent and mechanisms remain to be discovered.
Other Microbiomes and Mental Health
The majority of human microbiome interaction with regards to human health usually includes the gut to some degree, though there are some questions as to whether certain microbiomes create their own axes with the brain.
The oral microbiome can potentially get direct or indirect access to the brain through the olfactory tract, or via the circulatory system where blood can transport microbes to the blood-brain barrier (BBB), perivascular spaces, and circumventricular organs (Figure 4) (Olsen and Singhrao, 2015, Ranjan et al., 2018, Olsen and Hicks, 2019). Microbial access to the bloodstream can commonly occur during dental procedures, and pathogen invasion of the brain can impact neuro-immune activity and inflammation (Olsen, 2008). These oral-brain axis-derived infections could contribute to mental disorders such as Alzheimer’s disease (AD), dementia, Down’s syndrome, bipolar disorder, and autism spectrum disorder (ASD; which can lead to oral dysbiosis) (Ilievski et al., 2018, Olsen and Hicks, 2019, Maitre et al., 2020). Oral dysbiosis can also promote the development of ASD by affecting the metabolome and thus can create a troublesome positive feedback loop (Mussap et al., 2016, Wang et al., 2016).
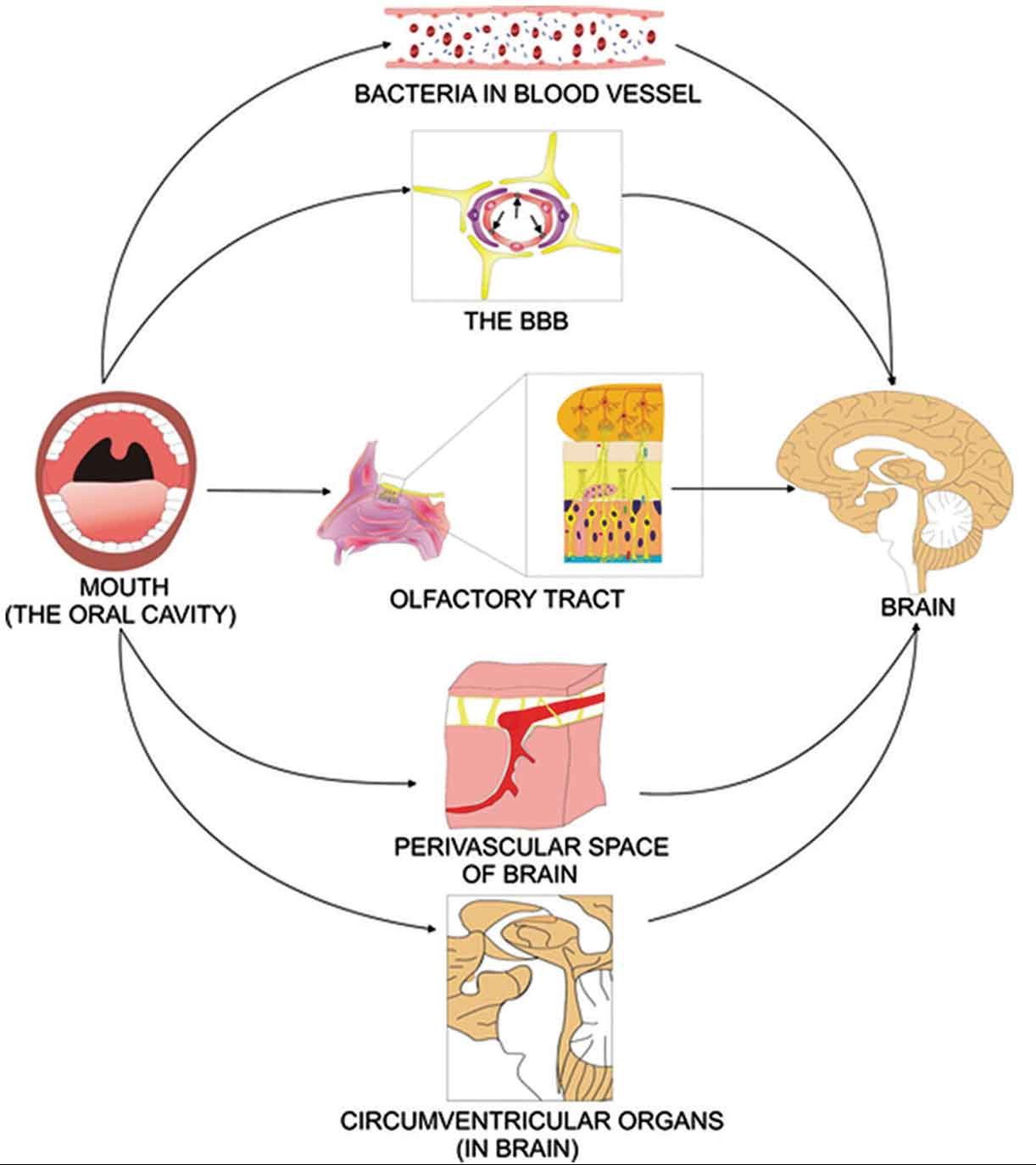
Concerning the skin-brain axis, dysbiosis of the skin microbiome and chronic wounds can elicit systemic effects and induce neural responses that eventually affect the central nervous system (Figure 5) (Hadian et al., 2020). Chronic wounds exhibit persistent inflammation and can have various etiologies including diabetic foot ulcers (DFU), venous ulcers, arterial ulcers, pressure ulcers, surgical wounds, and other traumatic wounds (Green et al., 2014, Renner and Erfurt-Berge, 2017, Bui et al., 2018, Pedras et al., 2018, Hadian et al., 2020). In these cases, a compromised skin barrier can allow pathogens and their derivatives to enter the bloodstream, and if the skin is in a dysbiotic state, then abundant pathogens such as Staphylococcus aureus and Pseudomonas aeruginosa can amplify this effect by inducing epithelial permeability (Roy et al., 2014, Basler et al., 2017). These microbes, metabolites, pro-inflammatory mediators, and other constituents circulate within the blood, potentially induce permeability in the blood-brain barrier (BBB), and eventually reach the brain to cause disorders such as wound-associated depression, anxiety, and other cognitive disorders (Wang et al., 2011, Zhang et al., 2015, Hadian et al., 2020). The skin-brain axis acts in a bi-direction manner too, with studies showing connections between certain mental disorders like post-traumatic stress disorder, and skin diseases associated with dysbiosis like psoriasis, chronic urticaria (hives), and atopic dermatitis (Gupta et al., 2017, Beri, 2018).
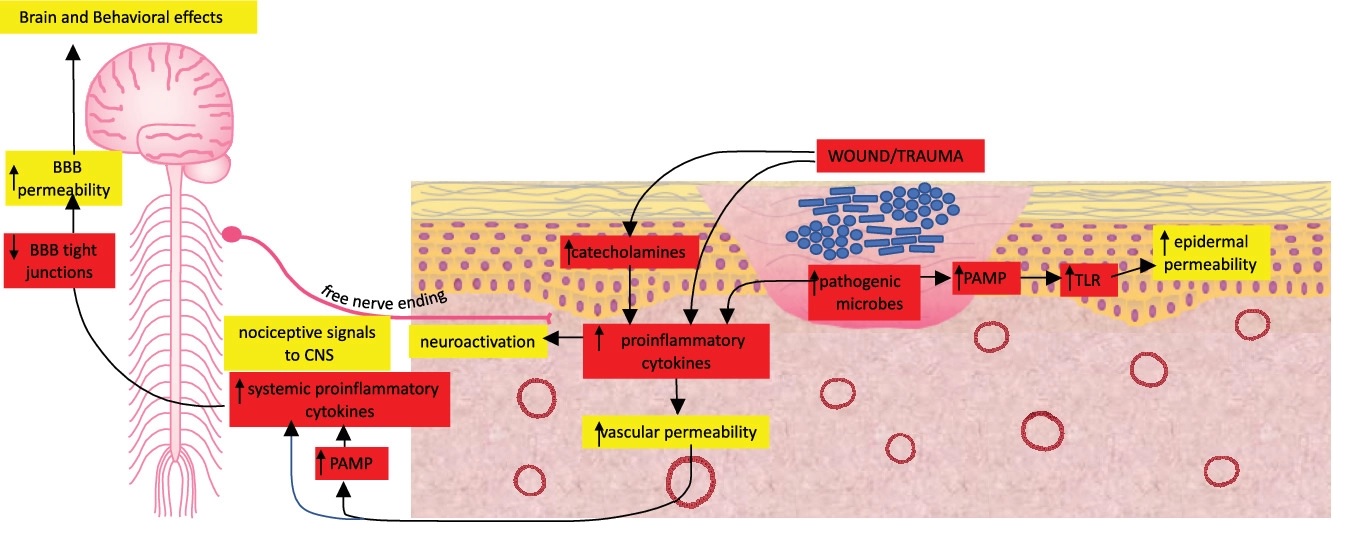
The lung-brain axis connects pulmonary microbes to neurodegenerative disorders and behavior characteristics, and though not much research has focused on this specific link, there are indications that air pollution can be a trigger. Pollutants in the air come from a variety of sources including engine emissions, coal combustion, biomass burning, and secondary photochemical products, such as ground level ozone (O3) (Mumaw et al., 2016). These compounds can be a source of chronic neuroinflammation and persistently affect microglial cells in the central nervous system, which in turn can increase risk of diseases such as Alzheimer’s disease, Parkinson’s disease, and autism, as well as elicit a decline in cognitive function in the elderly (Power et al., 2011, Wellenius et al., 2012, Roberts et al., 2013, Volk et al., 2013, Heneka et al., 2014, Jung et al., 2015, Kirrane et al., 2015, Mumaw et al., 2016). It is likely that air pollution also negatively affects the lung microbiome, and dysbiosis could further aggravate immune responses and cognitive disruption (Mousavi et al., 2021, Whiteside et al., 2021).
Is there a brain microbiome?
That is, are there resident microbes residing with a living brain? With many neurodegenerative and neuropsychiatric diseases lacking a clear etiology, determining any and all potential associations could help in therapeutic efforts (Link, 2021). The healthy brain is an assumedly sterile environment, though the same was once thought about other organs like the lungs. Interestingly, one study found evidence for the existence of viable bacteria in the human brain. Here, researchers were interested to see whether microbial invasion accompanied damaged brains observed in HIV/AIDS. After sequencing total RNA from cerebral white matter, they found bacteria and phage sequences in both experimental and control brain samples, which was further validated by 16S rRNA gene target amplification and in situ staining (Branton et al., 2013, Link, 2021).
If there were resident microbes in the brain, they would certainly be at much lower abundances as compared with other regions such as the gut or the oral cavity. Though there has been much interest in characterizing the presence of pathogens in unhealthy brains for disorders like Alzheimer’s disease, which is an arduous feat in itself, finding residents in a healthy state could prove even more difficult (Zhan et al., 2016, Alonzo et al., 2018, Dominy et al., 2019, Link, 2021). That is, identification approaches could easily miss novel or fastidious microorganisms, and contamination is hard to avoid. Furthermore, a wide-range of controlled and unbiased studies are necessary to catalogue a possible brain microbiome, however, completing this task in humans brings about ethical implications and sampling limitations (Link, 2021). It is fascinating to consider the prospect of symbiotic brain microorganisms, and how they could influence our health or who we are.
Conclusion
Mental faculties contribute greatly to human health, and therefore characterizing the involvement of the human microbiome is of substantial importance. Many of these select microbiomes overlap, and the disorders associated with each are likely entangled in a complex array. Brain functions are extremely complex as is, and piecing together the microbial puzzle within their reach further complicates matters. However, there is great optimism in considering the human microbiome as a source or target for advanced therapy to resolve mental disorders and other diseases, which could drastically change the way we think about medicine.
Discussion Concept Mapping
With a partner or as a class, connect two or more microbiomes using a concept map. Explain how and/or why the various microbiomes are linked (e.g., disease cause vs. effect, direct vs. indirect, pathways, consortia translocation, factors affecting composition, etc.).
Check your Understanding
- In what ways does the gut microbiome connect with other microbiomes and regions of the body? How does it influence the development of diseases in these areas?
- How could early use of antibiotics influence the development of asthma in children?
- How could gut microbiome metabolites (SCFAs) contribute to healthy human states?
- In what ways does the human microbiome communicate with the brain?
- What options are available to treat mental disorders through the gut microbiome?
- Why have mental illnesses been increasing in prevalence and how could the gut microbiome be involved?
- How are microbiomes, other than the gut, implicated in mental health?
- What role does skin microbiome dysbiosis and chronic wounds have in the development of cognitive disorders?
Media Attributions
- Figure 1 – Inflammatory and microbial influences between the gut and skin for a healthy state by De Pessemier et al., 2021. Licensed under the Creative Commons Attribution (CC BY) license (http://creativecommons.org/licenses/by/4.0/).
- Video 1 – Remodeling of the maternal gut microbiome during pregnancy is shaped by parity by Research Square. Licensed under Creative Commons: By Attribution 3.0 License https://creativecommons.org/licenses/by/3.0/
- Figure 2 – Gut-brain interactions with mental health by Bear et al., 2021. Licensed under the Creative Commons Attribution (CC BY) license (http://creativecommons.org/licenses/by/4.0/).
- Figure 3 – Development of the gut-brain over time by Liang et al., 2018. Licensed under the Creative Commons Attribution (CC BY) license (http://creativecommons.org/licenses/by/4.0/).
- Video 2 – Functional GI Disorders: The role of the gut-brain interaction, microbiome and nutrition by UMMCVideos. Licensed under Creative Commons Attribution license (reuse allowed).
-
Figure 4 – Direct and indirect mechanisms of infecting the brain by Olsen and Hicks, 2019 licensed under under the terms of the Creative Commons Attribution License (http://creativecommons.org/licenses/by/4.0
- Figure 5 – Chronic wounds and skin-brain axis by Hadian et al., 2020 under Public Domain
References
- Acharya, C., Sahingur, S. E., & Bajaj, J. S. (2017). Microbiota, cirrhosis, and the emerging oral-gut-liver axis. JCI Insight, 2(19), e94416. https://doi.org/10.1172/jci.insight.94416
- Allali, I., Bakri, Y., Amzazi, S., & Ghazal, H. (2021). Gut-Lung Axis in COVID-19. Interdisciplinary Perspectives on Infectious Diseases, 2021, 6655380. https://doi.org/10.1155/2021/6655380
- Alonso, R., Pisa, D., Fernández-Fernández, A. M., & Carrasco, L. (2018). Infection of Fungi and Bacteria in Brain Tissue From Elderly Persons and Patients With Alzheimer’s Disease. Frontiers in Aging Neuroscience, 10. https://www.frontiersin.org/article/10.3389/fnagi.2018.00159
- Bäsler, K., Galliano, M.-F., Bergmann, S., Rohde, H., Wladykowski, E., Vidal-y-Sy, S., Guiraud, B., Houdek, P., Schüring, G., Volksdorf, T., Caruana, A., Bessou-Touya, S., Schneider, S. W., Duplan, H., & Brandner, J. M. (2017). Biphasic influence of Staphylococcus aureus on human epidermal tight junctions. Annals of the New York Academy of Sciences, 1405(1), 53–70. https://doi.org/https://doi.org/10.1111/nyas.13418
- Bear T, Dalziel J, Coad J, Roy N, Butts C, & Gopal P. (2021). The Microbiome-Gut-Brain Axis and Resilience to Developing Anxiety or Depression under Stress. Microorganisms, 9(4):723. https://doi.org/10.3390/microorganisms9040723
- Beri, K. (2018). Skin microbiome & host immunity: applications in regenerative cosmetics & transdermal drug delivery. Future Science OA, 4(6), FSO302. https://doi.org/10.4155/fsoa-2017-0117
- Boix-Amorós, A., Collado, M. C., & Mira, A. (2016). Relationship between Milk Microbiota, Bacterial Load, Macronutrients, and Human Cells during Lactation. Frontiers in microbiology, 7, 492. https://doi.org/10.3389/fmicb.2016.00492
- Borre, Y. E., O’Keeffe, G. W., Clarke, G., Stanton, C., Dinan, T. G., & Cryan, J. F. (2014). Microbiota and neurodevelopmental windows: implications for brain disorders. Trends in Molecular Medicine, 20(9), 509–518. https://doi.org/10.1016/j.molmed.2014.05.002
- Branton, W. G., Ellestad, K. K., Maingat, F., Wheatley, B. M., Rud, E., Warren, R. L., Holt, R. A., Surette, M. G., & Power, C. (2013). Brain Microbial Populations in HIV/AIDS: α-Proteobacteria Predominate Independent of Host Immune Status. PLOS ONE, 8(1), e54673-. https://doi.org/10.1371/journal.pone.0054673
- Bruce-Keller, A. J., Salbaum, J. M., & Berthoud, H.-R. (2018). Harnessing Gut Microbes for Mental Health: Getting From Here to There. Biological Psychiatry, 83(3), 214–223. https://doi.org/10.1016/j.biopsych.2017.08.014
- Bui, U. T., Finlayson, K., & Edwards, H. (2018). Risk factors for infection in patients with chronic leg ulcers: A survival analysis. International Journal of Clinical Practice, 72(12), e13263. https://doi.org/10.1111/ijcp.13263
- Carlson, A. L., Xia, K., Azcarate-Peril, M. A., Goldman, B. D., Ahn, M., Styner, M. A., Thompson, A. L., Geng, X., Gilmore, J. H., & Knickmeyer, R. C. (2018). Infant Gut Microbiome Associated With Cognitive Development. Biological Psychiatry, 83(2), 148–159. https://doi.org/10.1016/j.biopsych.2017.06.021
- Civardi, E., Garofoli, F., Tzialla, C., Paolillo, P., Bollani, L., & Stronati, M. (2013). Microorganisms in human milk: lights and shadows. The journal of maternal-fetal & neonatal medicine : the official journal of the European Association of Perinatal Medicine, the Federation of Asia and Oceania Perinatal Societies, the International Society of Perinatal Obstetricians, 26 Suppl 2, 30–34. https://doi.org/10.3109/14767058.2013.829693
- Cowan, C. S. M., Hoban, A. E., Ventura-Silva, A. P., Dinan, T. G., Clarke, G., & Cryan, J. F. (2018). Gutsy Moves: The Amygdala as a Critical Node in Microbiota to Brain Signaling. BioEssays, 40(1), 1700172. https://doi.org/https://doi.org/10.1002/bies.201700172
- Cryan, J. F., & Dinan, T. G. (2012). Mind-altering microorganisms: the impact of the gut microbiota on brain and behaviour. Nature Reviews Neuroscience, 13(10), 701–712. https://doi.org/10.1038/nrn3346
- Cryan, J. F., O’Riordan, K. J., Cowan, C. S. M., Sandhu, K. v, Bastiaanssen, T. F. S., Boehme, M., Codagnone, M. G., Cussotto, S., Fulling, C., Golubeva, A. v, Guzzetta, K. E., Jaggar, M., Long-Smith, C. M., Lyte, J. M., Martin, J. A., Molinero-Perez, A., Moloney, G., Morelli, E., Morillas, E., … Dinan, T. G. (2019). The Microbiota-Gut-Brain Axis. Physiological Reviews, 99(4), 1877–2013. https://doi.org/10.1152/physrev.00018.2018
- Dash, S., Clarke, G., Berk, M., & Jacka, F. N. (2015). The gut microbiome and diet in psychiatry: focus on depression. Current Opinion in Psychiatry, 28(1). https://journals.lww.com/co-psychiatry/Fulltext/2015/01000/The_gut_microbiome_and_diet_in_psychiatry__focus.2.aspx
- De Pessemier B, Grine L, Debaere M, Maes A, Paetzold B, Callewaert C. (2021). Gut–Skin Axis: Current Knowledge of the Interrelationship between Microbial Dysbiosis and Skin Conditions. Microorganisms, 9(2):353. https://doi.org/10.3390/microorganisms9020353
- Desbonnet, L., Clarke, G., Shanahan, F., Dinan, T. G., & Cryan, J. F. (2014). Microbiota is essential for social development in the mouse. Molecular Psychiatry, 19(2), 146–148. https://doi.org/10.1038/mp.2013.65
- Diaz Heijtz, R. (2016). Fetal, neonatal, and infant microbiome: Perturbations and subsequent effects on brain development and behavior. Seminars in Fetal and Neonatal Medicine, 21(6), 410–417. https://doi.org/10.1016/j.siny.2016.04.012
- Dinan, T. G., & Cryan, J. F. (2017). Gut instincts: microbiota as a key regulator of brain development, ageing and neurodegeneration. The Journal of Physiology, 595(2), 489–503. https://doi.org/10.1113/JP273106
- Dinan, T. G., Stanton, C., & Cryan, J. F. (2013). Psychobiotics: A Novel Class of Psychotropic. Biological Psychiatry, 74(10), 720–726. https://doi.org/10.1016/j.biopsych.2013.05.001
- Dominy, S. S., Casey, L., Florian, E., Malgorzata, B., Agata, M., Andrei, K., Mai, N., Ursula, H., Debasish, R., Christina, G., J, H. L., Shirin, A.-K., Samer, K., Alexander, L., I, R. M., Barbara, P., Piotr, M., Annelie, H., Karina, A., … Jan, P. (2022). Porphyromonas gingivalis in Alzheimer’s disease brains: Evidence for disease causation and treatment with small-molecule inhibitors. Science Advances, 5(1), eaau3333. https://doi.org/10.1126/sciadv.aau3333
- Drago, L., Zuccotti, G. V., Romanò, C. L., Goswami, K., Villafañe, J. H., Mattina, R., & Parvizi, J. (2019). Oral–Gut Microbiota and Arthritis: Is There an Evidence-Based Axis? Journal of Clinical Medicine, 8(10):1753. https://doi.org/10.3390/jcm8101753
- du Teil Espina, M., Gabarrini, G., Harmsen, H. J. M., Westra, J., van Winkelhoff, A. J., & van Dijl, J. M. (2019). Talk to your gut: the oral-gut microbiome axis and its immunomodulatory role in the etiology of rheumatoid arthritis. FEMS Microbiology Reviews, 43(1), 1–18. https://doi.org/10.1093/femsre/fuy035
- Enaud, R., Prevel, R., Ciarlo, E., Beaufils, F., Wieërs, G., Guery, B., & Delhaes, L. (2020). The Gut-Lung Axis in Health and Respiratory Diseases: A Place for Inter-Organ and Inter-Kingdom Crosstalks. Frontiers in Cellular and Infection Microbiology, 10. https://www.frontiersin.org/article/10.3389/fcimb.2020.00009
- Frati F, Salvatori C, Incorvaia C, Bellucci A, Di Cara G, Marcucci F, & Esposito S. (2019). The Role of the Microbiome in Asthma: The Gut–Lung Axis. International Journal of Molecular Sciences, 20(1):123. https://doi.org/10.3390/ijms20010123
- Gareau, M. G. (2016). Chapter Eleven – Cognitive Function and the Microbiome. In J. F. Cryan & G. Clarke (Eds.), International Review of Neurobiology (Vol. 131, pp. 227–246). Academic Press. https://doi.org/10.1016/bs.irn.2016.08.001
- Green, J., Jester, R., McKinley, R., & Pooler, A. (2014). The impact of chronic venous leg ulcers: a systematic review. Journal of Wound Care, 23(12), 601–612. https://doi.org/10.12968/jowc.2014.23.12.601
- Gupta, M. A., Jarosz, P., & Gupta, A. K. (2017). Posttraumatic stress disorder (PTSD) and the dermatology patient. Clinics in Dermatology, 35(3), 260–266. https://doi.org/10.1016/j.clindermatol.2017.01.005
- Gur, T. L., Worly, B. L., & Bailey, M. T. (2015). Stress and the Commensal Microbiota: Importance in Parturition and Infant Neurodevelopment. Frontiers in Psychiatry, 6. https://www.frontiersin.org/article/10.3389/fpsyt.2015.00005
- Hassan, Z., Mustafa, S., Rahim, R. A., & Isa, N. M. (2016). Anti-breast cancer effects of live, heat-killed and cytoplasmic fractions of Enterococcus faecalis and Staphylococcus hominis isolated from human breast milk. In vitro cellular & developmental biology. Animal, 52(3), 337–348. https://doi.org/10.1007/s11626-015-9978-8
- He, Z., Cui, B.-T., Zhang, T., Li, P., Long, C.-Y., Ji, G.-Z., & Zhang, F.-M. (2017). Fecal microbiota transplantation cured epilepsy in a case with Crohn’s disease: The first report. World Journal of Gastroenterology, 23(19), 3565–3568. https://doi.org/10.3748/wjg.v23.i19.3565
- Heikkilä, M. P., & Saris, P. E. (2003). Inhibition of Staphylococcus aureus by the commensal bacteria of human milk. Journal of applied microbiology, 95(3), 471–478. https://doi.org/10.1046/j.1365-2672.2003.02002.x
- Heneka, M. T., Kummer, M. P., & Latz, E. (2014). Innate immune activation in neurodegenerative disease. Nature Reviews Immunology, 14(7), 463–477. https://doi.org/10.1038/nri3705
- Hoban, A. E., Stilling, R. M., Moloney, G., Shanahan, F., Dinan, T. G., Clarke, G., & Cryan, J. F. (2018). The microbiome regulates amygdala-dependent fear recall. Molecular Psychiatry, 23(5), 1134–1144. https://doi.org/10.1038/mp.2017.100
- Ilievski, V., Zuchowska, P. K., Green, S. J., Toth, P. T., Ragozzino, M. E., Le, K., Aljewari, H. W., O’Brien-Simpson, N. M., Reynolds, E. C., & Watanabe, K. (2018). Chronic oral application of a periodontal pathogen results in brain inflammation, neurodegeneration and amyloid beta production in wild type mice. PLOS ONE, 13(10), e0204941-. https://doi.org/10.1371/journal.pone.0204941
- Jung, C.-R., Lin, Y.-T., & Hwang, B.-F. (2015). Ozone, Particulate Matter, and Newly Diagnosed Alzheimer’s Disease: A Population-Based Cohort Study in Taiwan. Journal of Alzheimer’s Disease, 44, 573–584. https://doi.org/10.3233/JAD-140855
- Kang, D.-W., Adams, J. B., Gregory, A. C., Borody, T., Chittick, L., Fasano, A., Khoruts, A., Geis, E., Maldonado, J., McDonough-Means, S., Pollard, E. L., Roux, S., Sadowsky, M. J., Lipson, K. S., Sullivan, M. B., Caporaso, J. G., & Krajmalnik-Brown, R. (2017). Microbiota Transfer Therapy alters gut ecosystem and improves gastrointestinal and autism symptoms: an open-label study. Microbiome, 5(1), 10. https://doi.org/10.1186/s40168-016-0225-7
- Kelly, J., Kennedy, P., Cryan, J., Dinan, T., Clarke, G., & Hyland, N. (2015). Breaking Down the Barriers: The Gut Microbiome, Intestinal Permeability and Stress-related Psychiatric Disorders. Frontiers in Cellular Neuroscience, 9. https://www.frontiersin.org/article/10.3389/fncel.2015.00392
- Kesika, P., Suganthy, N., Sivamaruthi, B. S., & Chaiyasut, C. (2021). Role of gut-brain axis, gut microbial composition, and probiotic intervention in Alzheimer’s disease. Life Sciences, 264, 118627. https://doi.org/10.1016/j.lfs.2020.118627
- Kirrane, E. F., Bowman, C., Davis, J. A., Hoppin, J. A., Blair, A., Chen, H., Patel, M. M., Sandler, D. P., Tanner, C. M., Vinikoor-Imler, L., Ward, M. H., Luben, T. J., & Kamel, F. (2015). Associations of Ozone and PM2.5 Concentrations With Parkinson’s Disease Among Participants in the Agricultural Health Study. Journal of Occupational and Environmental Medicine, 57(5), 509–517. https://doi.org/10.1097/JOM.0000000000000451
- Kundu, P., Blacher, E., Elinav, E., & Pettersson, S. (2017). Our Gut Microbiome: The Evolving Inner Self. Cell, 171(7), 1481–1493. https://doi.org/10.1016/j.cell.2017.11.024
- Lara-Villoslada, F., Olivares, M., Sierra, S., Rodríguez, J. M., Boza, J., & Xaus, J. (2007). Beneficial effects of probiotic bacteria isolated from breast milk. The British journal of nutrition, 98 Suppl 1, S96–S100. https://doi.org/10.1017/S0007114507832910
- Leclercq, S., Cani, P. D., Neyrinck, A. M., Stärkel, P., Jamar, F., Mikolajczak, M., Delzenne, N. M., & de Timary, P. (2012). Role of intestinal permeability and inflammation in the biological and behavioral control of alcohol-dependent subjects. Brain, Behavior, and Immunity, 26(6), 911–918. https://doi.org/10.1016/j.bbi.2012.04.001
- Li, M., van Esch, B. C. A. M., Wagenaar, G. T. M., Garssen, J., Folkerts, G., & Henricks, P. A. J. (2018). Pro- and anti-inflammatory effects of short chain fatty acids on immune and endothelial cells. European Journal of Pharmacology, 831, 52–59. https://doi.org/10.1016/j.ejphar.2018.05.003
- Liang S, Wu X, Hu X, Wang T, & Jin F. (2018b). Recognizing Depression from the Microbiota–Gut–Brain Axis. International Journal of Molecular Sciences, 19(6):1592. https://doi.org/10.3390/ijms19061592
- Liang, S., Wang, T., Hu, X., Luo, J., Li, W., Wu, X., Duan, Y., & Jin, F. (2015). Administration of Lactobacillus helveticus NS8 improves behavioral, cognitive, and biochemical aberrations caused by chronic restraint stress. Neuroscience, 310, 561–577. https://doi.org/10.1016/j.neuroscience.2015.09.033
- Liang, S., Wu, X., & Jin, F. (2018). Gut-Brain Psychology: Rethinking Psychology From the Microbiota–Gut–Brain Axis. Frontiers in Integrative Neuroscience, 12. https://www.frontiersin.org/article/10.3389/fnint.2018.00033
- Limon, J. J., Tang, J., Li, D., Wolf, A. J., Michelsen, K. S., Funari, V., Gargus, M., Nguyen, C., Sharma, P., Maymi, V. I., Iliev, I. D., Skalski, J. H., Brown, J., Landers, C., Borneman, J., Braun, J., Targan, S. R., McGovern, D. P. B., & Underhill, D. M. (2019). Malassezia Is Associated with Crohn’s Disease and Exacerbates Colitis in Mouse Models. Cell Host & Microbe, 25(3), 377-388.e6. https://doi.org/10.1016/j.chom.2019.01.007
- Link, C. D. (2021). Is There a Brain Microbiome? Neuroscience Insights, 16, 26331055211018708–26331055211018708. https://doi.org/10.1177/26331055211018709
- Luczynski, P., McVey Neufeld, K.-A., Oriach, C. S., Clarke, G., Dinan, T. G., & Cryan, J. F. (2016). Growing up in a Bubble: Using Germ-Free Animals to Assess the Influence of the Gut Microbiota on Brain and Behavior. International Journal of Neuropsychopharmacology, 19(8), pyw020. https://doi.org/10.1093/ijnp/pyw020
- Ma, Q., Xing, C., Long, W., Wang, H. Y., Liu, Q., & Wang, R.-F. (2019). Impact of microbiota on central nervous system and neurological diseases: the gut-brain axis. Journal of Neuroinflammation, 16(1), 53. https://doi.org/10.1186/s12974-019-1434-3
- Maitre Y, Micheneau P, Delpierre A, Mahalli R, Guerin M, Amador G, Denis F. (2020). Did the Brain and Oral Microbiota Talk to Each Other? A Review of the Literature. Journal of Clinical Medicine, 9(12):3876. https://doi.org/10.3390/jcm9123876
- Manderino L, Carroll I, Azcarate-Peril MA, Rochette A, Heinberg L, Peat C, Steffen K, Mitchell J, Gunstad J. Preliminary Evidence for an Association Between the Composition of the Gut Microbiome and Cognitive Function in Neurologically Healthy Older Adults. J Int Neuropsychol Soc. 2017 Sep;23(8):700-705. doi: 10.1017/S1355617717000492. Epub 2017 Jun 23. PMID: 28641593; PMCID: PMC6111127.
- Mika, A., Day, H. E. W., Martinez, A., Rumian, N. L., Greenwood, B. N., Chichlowski, M., Berg, B. M., & Fleshner, M. (2017). Early life diets with prebiotics and bioactive milk fractions attenuate the impact of stress on learned helplessness behaviours and alter gene expression within neural circuits important for stress resistance. European Journal of Neuroscience, 45(3), 342–357. https://doi.org/https://doi.org/10.1111/ejn.13444
- Mika, A., Day, H. E. W., Martinez, A., Rumian, N. L., Greenwood, B. N., Chichlowski, M., Berg, B. M., & Fleshner, M. (2017). Early life diets with prebiotics and bioactive milk fractions attenuate the impact of stress on learned helplessness behaviours and alter gene expression within neural circuits important for stress resistance. European Journal of Neuroscience, 45(3), 342–357. https://doi.org/10.1111/ejn.13444
- Mousavi, S. E., Delgado-Saborit, J. M., Adivi, A., Pauwels, S., & Godderis, L. (2021). Air pollution and endocrine disruptors induce human microbiome imbalances: A systematic review of recent evidence and possible biological mechanisms. Science of The Total Environment, 151654. https://doi.org/10.1016/j.scitotenv.2021.151654
- Mumaw, C. L., Levesque, S., McGraw, C., Robertson, S., Lucas, S., Stafflinger, J. E., Campen, M. J., Hall, P., Norenberg, J. P., Anderson, T., Lund, A. K., McDonald, J. D., Ottens, A. K., & Block, M. L. (2016). Microglial priming through the lung—brain axis: the role of air pollution-induced circulating factors. The FASEB Journal, 30(5), 1880–1891. https://doi.org/10.1096/fj.201500047
- Mussap, M., Noto, A., & Fanos, V. (2016). Metabolomics of autism spectrum disorders: early insights regarding mammalian-microbial cometabolites. Expert Review of Molecular Diagnostics, 16(8), 869–881. https://doi.org/10.1080/14737159.2016.1202765
- Narengaowa, Kong, W., Lan, F., Awan, U. F., Qing, H., & Ni, J. (2021). The Oral-Gut-Brain AXIS: The Influence of Microbes in Alzheimer’s Disease. Frontiers in Cellular Neuroscience, 15. https://www.frontiersin.org/article/10.3389/fncel.2021.633735
- Nishida, A. H., & Ochman, H. (2018). Rates of gut microbiome divergence in mammals. Molecular Ecology, 27(8), 1884–1897. https://doi.org/10.1111/mec.14473
- Nyangahu, D. D., & Jaspan, H. B. (2019). Influence of maternal microbiota during pregnancy on infant immunity. Clinical & Experimental Immunology, 198(1), 47–56. https://doi.org/10.1111/cei.13331
- O’Mahony, S. M., Clarke, G., Dinan, T. G., & Cryan, J. F. (2017). Early-life adversity and brain development: Is the microbiome a missing piece of the puzzle? Neuroscience, 342, 37–54. https://doi.org/10.1016/j.neuroscience.2015.09.068
- O’Neill, C. A., Monteleone, G., McLaughlin, J. T., & Paus, R. (2016). The gut-skin axis in health and disease: A paradigm with therapeutic implications. BioEssays, 38(11), 1167–1176. https://doi.org/10.1002/bies.201600008
- Ojo-Okunola, A., Claassen-Weitz, S., Mwaikono, K. S., Gardner-Lubbe, S., Stein, D. J., Zar, H. J., Nicol, M. P., & du Toit, E. (2019) Influence of Socio-Economic and Psychosocial Profiles on the Human Breast Milk Bacteriome of South African Women. Nutrients, 11(6):1390. https://doi.org/10.3390/nu11061390
- Ojo-Okunola, A., Nicol, M., & Du Toit, E. (2018). Human Breast Milk Bacteriome in Health and Disease. Nutrients, 10(11):1643. https://doi.org/10.3390/nu10111643
- Olivares, M., Díaz-Ropero, M. P., Martín, R., Rodríguez, J. M., & Xaus, J. (2006). Antimicrobial potential of four Lactobacillus strains isolated from breast milk. Journal of applied microbiology, 101(1), 72–79. https://doi.org/10.1111/j.1365-2672.2006.02981.x
- Olsen, I. (2008). Update on bacteraemia related to dental procedures. Transfusion and Apheresis Science, 39(2), 173–178. https://doi.org/10.1016/j.transci.2008.06.008
- Olsen, I., & Hicks, S. D. (2020). Oral microbiota and autism spectrum disorder (ASD). Journal of Oral Microbiology, 12(1), 1702806. https://doi.org/10.1080/20002297.2019.1702806
- Olsen, I., & Singhrao, S. K. (2015). Can oral infection be a risk factor for Alzheimer’s disease? Journal of Oral Microbiology, 7(1), 29143. https://doi.org/10.3402/jom.v7.29143
- Park S-Y, Hwang B-O, Lim M, Ok S-H, Lee S-K, Chun K-S, Park K-K, Hu Y, Chung W-Y, Song N-Y. (2021). Oral–Gut Microbiome Axis in Gastrointestinal Disease and Cancer. Cancers, 13(9):2124. https://doi.org/10.3390/cancers13092124
- Pedras, S., Carvalho, R., & Pereira, M. G. (2016). Predictors of quality of life in patients with diabetic foot ulcer: The role of anxiety, depression, and functionality. Journal of Health Psychology, 23(11), 1488–1498. https://doi.org/10.1177/1359105316656769
- Penders, J., Thijs, C., van den Brandt, P. A., Kummeling, I., Snijders, B., Stelma, F., Adams, H., van Ree, R., & Stobberingh, E. E. (2007). Gut microbiota composition and development of atopic manifestations in infancy: the KOALA Birth Cohort Study. Gut, 56(5), 661. https://doi.org/10.1136/gut.2006.100164
- Pirbaglou, M., Katz, J., de Souza, R. J., Stearns, J. C., Motamed, M., & Ritvo, P. (2016). Probiotic supplementation can positively affect anxiety and depressive symptoms: a systematic review of randomized controlled trials. Nutrition Research, 36(9), 889–898. https://doi.org/10.1016/j.nutres.2016.06.009
- Potgieter, M., Bester, J., Kell, D. B., & Pretorius, E. (2015). The dormant blood microbiome in chronic, inflammatory diseases. FEMS Microbiology Reviews, 39(4), 567–591. https://doi.org/10.1093/femsre/fuv013
- Power, M. C., Weisskopf, M. G., Alexeeff, S. E., Coull, B. A., Spiro, A., & Swartz, J. (2011). Traffic-Related Air Pollution and Cognitive Function in a Cohort of Older Men. Environmental Health Perspectives, 119(5), 682–687. https://doi.org/10.1289/ehp.1002767
- Ranjan, R., Abhinay, A., & Mishra, M. (2018). Can oral microbial infections be a risk factor for neurodegeneration? A review of the literature. Neurol India, 66:344-51
- Renner R, Erfurt-Berge C. (2017). Depression and quality of life in patients with chronic wounds: ways to measure their influence and their effect on daily life. Chronic Wound Care Management and Research, 4:143-151 https://doi.org/10.2147/CWCMR.S124917
- Roberts, A. L., Kristen, L., Hart, J. E., Francine, L., Just, A. C., Bobb, J. F., Koenen, K. C., Alberto, A., & Weisskopf, M. G. (2013). Perinatal Air Pollutant Exposures and Autism Spectrum Disorder in the Children of Nurses’ Health Study II Participants. Environmental Health Perspectives, 121(8), 978–984. https://doi.org/10.1289/ehp.1206187
- Rook, G. A. W., & Lowry, C. A. (2008). The hygiene hypothesis and psychiatric disorders. Trends in Immunology, 29(4), 150–158. https://doi.org/10.1016/j.it.2008.01.002
- Roy, S., Elgharably, H., Sinha, M., Ganesh, K., Chaney, S., Mann, E., Miller, C., Khanna, S., Bergdall, V. K., Powell, H. M., Cook, C. H., Gordillo, G. M., Wozniak, D. J., & Sen, C. K. (2014). Mixed-species biofilm compromises wound healing by disrupting epidermal barrier function. The Journal of Pathology, 233(4), 331–343. https://doi.org/10.1002/path.4360
- Salem, I., Ramser, A., Isham, N., & Ghannoum, M. A. (2018). The Gut Microbiome as a Major Regulator of the Gut-Skin Axis. Frontiers in Microbiology, 9. https://www.frontiersin.org/article/10.3389/fmicb.2018.01459
- Salliss, M. E., Farland, L. v, Mahnert, N. D., & Herbst-Kralovetz, M. M. (2022). The role of gut and genital microbiota and the estrobolome in endometriosis, infertility and chronic pelvic pain. Human Reproduction Update, 28(1), 92–131. https://doi.org/10.1093/humupd/dmab035
- Sampson, T. R., & Mazmanian, S. K. (2015). Control of Brain Development, Function, and Behavior by the Microbiome. Cell Host & Microbe, 17(5), 565–576. https://doi.org/10.1016/j.chom.2015.04.011
- Sencio, V., Barthelemy, A., Tavares, L. P., Machado, M. G., Soulard, D., Cuinat, C., Queiroz-Junior, C. M., Noordine, M.-L., Salomé-Desnoulez, S., Deryuter, L., Foligné, B., Wahl, C., Frisch, B., Vieira, A. T., Paget, C., Milligan, G., Ulven, T., Wolowczuk, I., Faveeuw, C., … Trottein, F. (2020). Gut Dysbiosis during Influenza Contributes to Pulmonary Pneumococcal Superinfection through Altered Short-Chain Fatty Acid Production. Cell Reports, 30(9), 2934-2947.e6. https://doi.org/10.1016/j.celrep.2020.02.013
- Sharon, G., Sampson, T. R., Geschwind, D. H., & Mazmanian, S. K. (2016). The Central Nervous System and the Gut Microbiome. Cell, 167(4), 915–932. https://doi.org/10.1016/j.cell.2016.10.027
- Sinha, S., Lin, G., & Ferenczi, K. (2021). The skin microbiome and the gut-skin axis. Clinics in Dermatology, 39(5), 829–839. https://doi.org/10.1016/j.clindermatol.2021.08.021
- Slyepchenko, A., Maes, M., Jacka, F. N., Köhler, C. A., Barichello, T., McIntyre, R. S., Berk, M., Grande, I., Foster, J. A., Vieta, E., & Carvalho, A. F. (2017). Gut Microbiota, Bacterial Translocation, and Interactions with Diet: Pathophysiological Links between Major Depressive Disorder and Non-Communicable Medical Comorbidities. Psychotherapy and Psychosomatics, 86(1), 31–46. https://doi.org/10.1159/000448957
- Slykerman, R. F., Thompson, J., Waldie, K. E., Murphy, R., Wall, C., & Mitchell, E. A. (2017). Antibiotics in the first year of life and subsequent neurocognitive outcomes. Acta Paediatrica, 106(1), 87–94. https://doi.org/10.1111/apa.13613
- Smythies, L. E., & Smythies, J. R. (2014). Microbiota, the immune system, black moods and the brain—melancholia updated. Frontiers in Human Neuroscience, 8. https://www.frontiersin.org/article/10.3389/fnhum.2014.00720
- Strachan, D. P. (1989). Hay fever, hygiene, and household size. BMJ (Clinical Research Ed.), 299(6710), 1259–1260. https://doi.org/10.1136/bmj.299.6710.1259
- Stumpf, R. M., Wilson, B. A., Rivera, A., Yildirim, S., Yeoman, C. J., Polk, J. D., White, B. A., & Leigh, S. R. (2013). The primate vaginal microbiome: Comparative context and implications for human health and disease. American Journal of Physical Anthropology, 152(S57), 119–134. https://doi.org/10.1002/ajpa.22395
- Taghinezhad-S, S., Keyvani, H., Bermúdez-Humarán, L. G., Donders, G. G. G., Fu, X., & Mohseni, A. H. (2021). Twenty years of research on HPV vaccines based on genetically modified lactic acid bacteria: an overview on the gut-vagina axis. Cellular and Molecular Life Sciences, 78(4), 1191–1206. https://doi.org/10.1007/s00018-020-03652-2
- Vaughan, A., Frazer, Z. A., Hansbro, P. M., & Yang, I. A. (2019). COPD and the gut-lung axis: the therapeutic potential of fibre. Journal of Thoracic Disease, 11(Suppl 17), S2173–S2180. https://doi.org/10.21037/jtd.2019.10.40
- Volk, H. E., Lurmann, F., Penfold, B., Hertz-Picciotto, I., & McConnell, R. (2013). Traffic-Related Air Pollution, Particulate Matter, and Autism. JAMA Psychiatry, 70(1), 71–77. https://doi.org/10.1001/jamapsychiatry.2013.266
- Vuong, H. E., Yano, J. M., Fung, T. C., & Hsiao, E. Y. (2017). The Microbiome and Host Behavior. Annual Review of Neuroscience, 40(1), 21–49. https://doi.org/10.1146/annurev-neuro-072116-031347
- Walker, R. W., Clemente, J. C., Peter, I., & Loos, R. J. F. (2017). The prenatal gut microbiome: are we colonized with bacteria in utero? Pediatric Obesity, 12(S1), 3–17. https://doi.org/10.1111/ijpo.12217
- Walker, W. A., & Iyengar, R. S. (2015). Breast milk, microbiota, and intestinal immune homeostasis. Pediatric research, 77(1-2), 220–228. https://doi.org/10.1038/pr.2014.160
- Wang, H., Liang, S., Wang, M., Gao, J., Sun, C., Wang, J., Xia, W., Wu, S., Sumner, S. J., Zhang, F., Sun, C., & Wu, L. (2016). Potential serum biomarkers from a metabolomics study of autism. Journal of Psychiatry & Neuroscience : JPN, 41(1), 27–37. https://doi.org/10.1503/jpn.140009
- Wang, T., Sha, L., Li, Y., Zhu, L., Wang, Z., Li, K., Lu, H., Bao, T., Guo, L., Zhang, X., & Wang, H. (2020). Dietary α-Linolenic Acid-Rich Flaxseed Oil Exerts Beneficial Effects on Polycystic Ovary Syndrome Through Sex Steroid Hormones—Microbiota—Inflammation Axis in Rats. Frontiers in Endocrinology, 11. https://www.frontiersin.org/article/10.3389/fendo.2020.00284
- Wang, W., Lv, S., Zhou, Y., Fu, J., Li, C., & Liu, P. (2011). Tumor necrosis factor-α affects blood–brain barrier permeability in acetaminophen-induced acute liver failure. European Journal of Gastroenterology & Hepatology, 23(7). https://journals.lww.com/eurojgh/Fulltext/2011/07000/Tumor_necrosis_factor___affects_blood_brain.2.aspx
- Watson, R. L., de Koff, E. M., & Bogaert, D. (2019). Characterising the respiratory microbiome. European Respiratory Journal, 53(2), 1801711. https://doi.org/10.1183/13993003.01711-2018
- Wellenius, G. A., Boyle, L. D., Coull, B. A., Milberg, W. P., Gryparis, A., Schwartz, J., Mittleman, M. A., & Lipsitz, L. A. (2012). Residential Proximity to Nearest Major Roadway and Cognitive Function in Community-Dwelling Seniors: Results from the MOBILIZE Boston Study. Journal of the American Geriatrics Society, 60(11), 2075–2080. https://doi.org/https://doi.org/10.1111/j.1532-5415.2012.04195.x
- Whiteside, S. A., McGinniss, J. E., & Collman, R. G. (2021). The lung microbiome: progress and promise. The Journal of Clinical Investigation, 131(15). https://doi.org/10.1172/jci150473
- Yang, Y., Tian, J., & Yang, B. (2018). Targeting gut microbiome: A novel and potential therapy for autism. Life Sciences, 194, 111–119. https://doi.org/10.1016/j.lfs.2017.12.027
- Zhan, X., Stamova, B., Jin, L.-W., DeCarli, C., Phinney, B., & Sharp, F. R. (2016). Gram-negative bacterial molecules associate with Alzheimer disease pathology. Neurology, 87(22), 2324. https://doi.org/10.1212/WNL.0000000000003391
- Zhang, J., Sadowska, G. B., Chen, X., Park, S. Y., Kim, J.-E., Bodge, C. A., Cummings, E., Lim, Y.-P., Makeyev, O., Besio, W. G., Gaitanis, J., Banks, W. A., & Stonestreet, B. S. (2015). Anti-IL-6 neutralizing antibody modulates blood-brain barrier function in the ovine fetus. The FASEB Journal, 29(5), 1739–1753.https://doi.org/10.1096/fj.14-258822